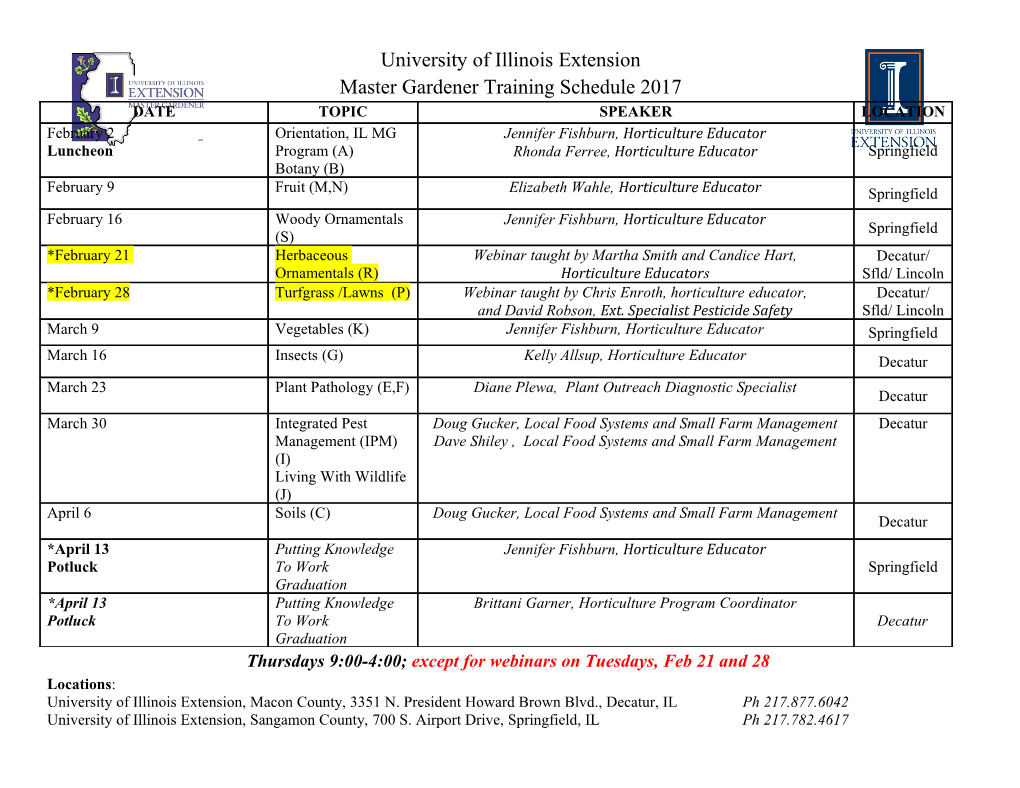
ENGINEERING SEMICONDUCTOR NANOCRYSTALS FOR MOLECULAR, CELLULAR, AND IN VIVO IMAGING A Dissertation Presented to The Academic Faculty By Andrew Michael Smith In Partial Fulfillment of the Requirements for the Degree Doctor of Philosophy in the Department of Biomedical Engineering Georgia Institute of Technology December, 2008 Copyright © 2008 by Andrew Michael Smith ENGINEERING SEMICONDUCTOR NANOCRYSTALS FOR MOLECULAR, CELLULAR, AND IN VIVO IMAGING Approved by: Dr. Shuming Nie, Advisor Dr. Zhong L. Wang School of Biomedical Engineering School of Materials Science and Georgia Institute of Technology Engineering Georgia Institute of Technology Dr. Gang Bao School of Biomedical Engineering Dr. Lily Yang Georgia Institute of Technology Department of Surgery Emory University Dr. Niren Murthy School of Biomedical Engineering Georgia Institute of Technology Date approved: October 16, 2008 ACKNOWLEDGEMENTS I dedicate this thesis to my parents, Marge and Winston, and my brother Edward, who have always supported me. I owe to them my values, work ethic, and aspiration to pursue a life of continuous learning. I thank my beautiful and incredibly intelligent girlfriend Mia for being compassionate and patient through the completion of my degree. I am grateful for guidance and inspiration from my friends, family members, and teachers, especially George Petsch, France Dorman, Mark Sommerville, James Powers, Athanassios Sambanis, Joseph Berglund, Schiue-Cheng Tang, Jonathan Beech, and James Mickens. I also thank Matthew Rhyner, Aaron Mohs, Michael Mancini, and Brad Kairdolf, who have taken part in the collaborative efforts of this thesis. Finally, I thank my research advisor, Shuming Nie, an inspiring leader and a brilliant mind who has given me tremendous opportunities over the past six years to learn a broad range of scientific disciplines, the encouragement to work harder than I have ever thought possible, and a vision for my future and the future of science and innovation. iii TABLE OF CONTENTS ACKNOWLEDGEMENTS . iii LIST OF TABLES . ix LIST OF FIGURES . x SUMMARY . xx CHAPTER 1 INTRODUCTION . 1 1.1 Thesis goals . 2 1.2 Themes and significant findings. 3 1.3 References . 5 2 SEMICONDUCTOR NANOCRYSTALS AND BIOLOGICAL APPLICATIONS 7 2.1 Semiconductor physics. 8 2.1.1 The electronic bandgap . 8 2.1.2 Absorption and luminescence. 11 2.1.3 Direct and indirect bandgaps . 12 2.1.4 Effective mass . 15 2.1.5 Excitons . 16 2.1.6 Semiconductor materials . 17 2.1.7 Chemical trends . 19 2.1.8 Band offsets . 26 2.2 Physics of semiconductor nanocrystals. 27 2.2.1 Quantum confinement . 27 2.2.2 Brus model of quantum confinement . 30 iv 2.2.3 Optical properties of quantum dots . 32 2.2.4 Surface properties of quantum dots . 36 2.2.5 Bandgap engineering . 41 2.3 Chemical synthesis of semiconductor nanocrystals . 44 2.3.1 Early quantum dot synthesis methods . 44 2.3.2 Coordinating solvent synthesis . 44 2.3.3 Heterostructure growth . 47 2.3.4 Engineering of quantum dot bioimaging probes . 48 2.4 Biological applications of semiconductor nanocrystals . 58 2.4.1 Comparison with conventional fluorophores . 61 2.4.2 In vitro diagnostic assays . 64 2.4.3 Cellular labeling . 69 2.4.4 In vivo animal imaging . 77 2.4.5 Quantum dot toxicity . 84 2.4.6 Outlook . 89 2.5 References . 91 3 QUANTUM DOT BANDGAP ENGINEERING VIA CATION EXCHANGE . 114 3.1 Synthesis methods . 115 3.2 Synthesis strategy . 120 3.3 Optical properties of cadmium mercury telluride nanocrystals . 123 3.4 Solvent impact on mercury cation exchange . 132 3.5 Mercury exchange with other II-VI materials . 135 3.6 Cation exchange mechanism . 137 3.7 Graded shell growth . 141 3.8 Outlook . 145 3.9 References . 147 v 4 QUANTUM DOT SHELL GROWTH AND THE IMPACT OF STRAIN . 150 4.1 Lattice strain in nanocrystals . 151 4.2 Colloidal synthesis methods for strained (core)shell nanocrystals . 156 4.3 Optical properties of strained (core)shell quantum dots . 162 4.4 Structural characterization of strained heterostructures . 176 4.5 Modeling of strain and band structures . 192 4.6 Other structural and quantum mechanical mechanisms . 198 4.7 Outlook . 201 4.8 References . 204 5 QUANTUM DOT SURFACE MODIFICATION AND SIZE MINIMIZATION . 210 5.1 Comparison of ligand and polymer coatings . 211 5.1.1 Experimental methods . 214 5.1.2 Optical and colloidal characterization . 217 5.1.3 Optical and structural stability assays . 221 5.1.4 Self-assembly and nanoparticle interface theory . 233 5.1.5 Selection of surface coatings for specific applications. 235 5.2 Quantum dot size minimization . 235 5.2.1 Size minimization of quantum dot micelles . 236 5.2.2 Size minimization using multivalent ligands . 241 5.2.2.1 Methods . 243 5.2.2.2 Multidentate ligand assembly . 253 5.2.2.3 Molar capping ratio . 257 5.2.2.4 Minimum size . 260 5.2.2.5 Stability . 261 5.2.2.6 Size comparison with proteins . 262 5.2.2.7 Outlook . 263 vi 5.3 References . 265 6 AMPHIPHILIC MULTIDENTATE LIGAND COATINGS . 270 6.1 Nanocrystal synthesis methods . 271 6.2 Hydrophilic and hydrophobic coatings in an amphibious bath . 276 6.2.1 Colloidal characterization . 278 6.2.2 Polymer shell characterization . 281 6.2.3 Reaction mechanism . 284 6.2.4 Materials compositions . 286 6.2.5 Applications of amphibious nanocrystals . 290 6.3 Control of nanocrystal growth, nucleation, and structure . 291 6.3.1 Reaction kinetics . 292 6.3.2 Lattice structure and morphology . 301 6.3.3 (Core)shell nanocrystal synthesis . 302 6.3.4 CdTe fluorescence stabilization . 305 6.4 Outlook . 308 6.5 References . 310 7 BIOCOMPATIBILITY OF QUANTUM DOTS . 314 7.1 Nonspecific binding to proteins . 315 7.1.1 Quantum dot surface coatings . 316 7.1.2 Quantum dot size and charge . 318 7.1.3 Mechanism of size-dependent protein adsorption . 323 7.2 Cellular binding and uptake of quantum dots . 329 7.2.1 Protocols . 329 7.2.2 Quantum dot charge effects . 330 7.2.3 Quantum dot size effects . 332 7.3 Bioconjugation to polyhistidine-tagged proteins . 339 vii 7.3.1 Quantum dot-protein A conjugation . 340 7.3.2 Quantum dot-protein A-IgG conjugation . 345 7.3.3 Optical properties of quantum dot-protein conjugates . 348 7.4 Quantum dot cytotoxicity . 353 7.4.1 Methods . 354 7.4.2 Cytotoxicity of reduced-cadmium quantum dots . 355 7.4.3 Cytotoxicity of core quantum dots. ..
Details
-
File Typepdf
-
Upload Time-
-
Content LanguagesEnglish
-
Upload UserAnonymous/Not logged-in
-
File Pages417 Page
-
File Size-