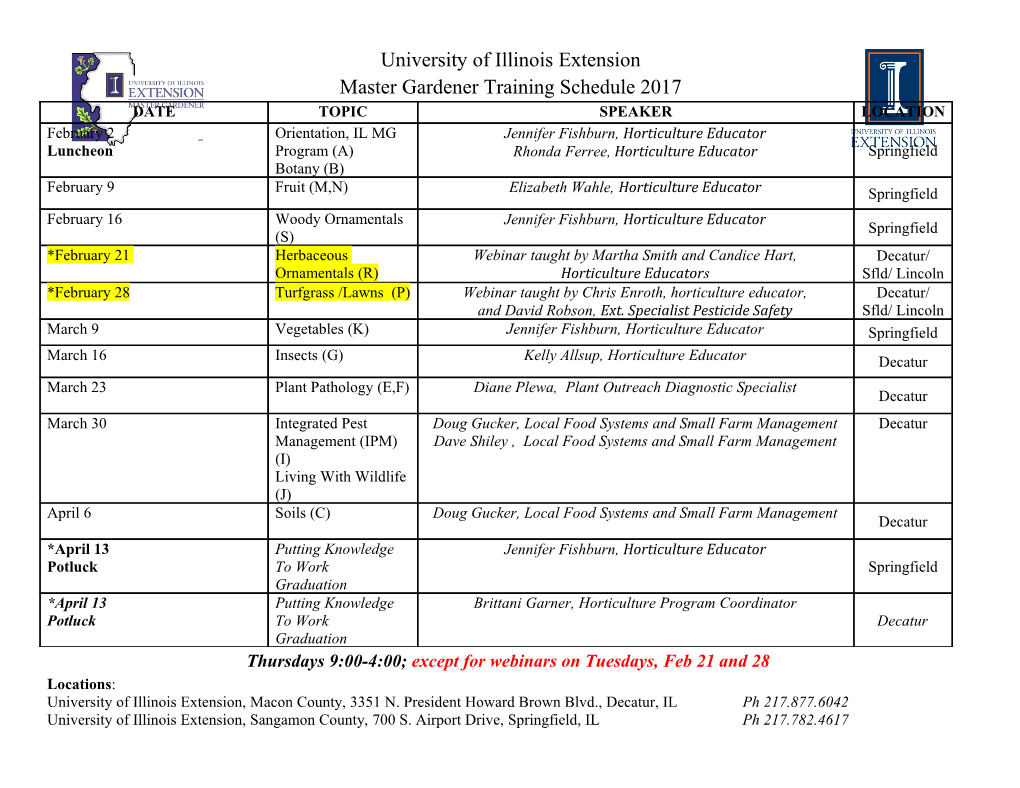
CHAPTER 4 Ionic basis of resting and action potentials Department of Physiology and Biophysics, University of Washington BERTIL HILLE I School of Medicine, Seattle, Washington CHAPTER CONTENTS Diffusion of charged particles in electric field Nernst-Planck equations Goldman-Hodgkin-Katz equation Development of Membrane Theory Ussing flux ratio Before intracellular recording Origins Independence relation Stimulation Eyring rate theory Conduction Practical calculations Cable Impedance First intracellular recordings from squid giant axons Resting potential Action potential EXCITABLE CELLS like other cells are completely sur- Afterpotential rounded by a thin unit membrane. Excitation of ex- Direct Measurement of Ionic Currents in Axon Membranes citable cells is always accompanied by changes in the Voltage-clamp method membrane potential. These changes may be abrupt Electrochemical separation of ionic currents Ion substitution experiments or slow, brief or long lasting, depending on the cell Reversal of current at Nernst potential and the stimulus, but the mechanisms of potential Tracer flux measurement change are fundamentally the same. They always Potassium current involve a change in the permeability of the mem- Pharmacological separation of ionic currents brane to ions. The guiding principles, called the Selective block Separate channels membrane theory or ionic hypothesis, may be stated Hodgkin-Huxley Model as follows: potentials a) develop across unit mem- Quantitative analysis ofl,,, and I, branes, b) depend on ionic concentration differences, Ionic conductances and c) arise because the membrane is selectively Hodgkin-Huxley equations Calculations with Hodgkin-Huxley model permeable to some ions. A corollary of these princi- Variety of Excitable Cells ples is that electric current flowing across mem- Myelinahd nerve branes is carried by the movement of ions. This chap- Saltatory conduction ter describes the electric phenomena of excitability, Ionic basis particularly as seen in axons, and shows how the Other axons Cell bodies potentials and currents at rest and during activity Gastropod ganglia may be analyzed and explained by the ionic hypothe- Vertebrate cell bodies sis. The approach is first historical, tracing the early Muscle development and first tests of the ionic hypothesis on Ionic Channels Sodium channels squid giant axons, then broadens to deal with the Number of channels modern consolidation of facts in many tissues, and Ionic selectivity concludes with derivations of some of the fundamen- Gating tal equations used to describe membrane potentials Potassium channels Long pore and ion fluxes. Some familiarity with classic proper- Ionic selectivity ties of excitable cells and the cable properties of axons Gating is assumed (see the chapter by Rall in this Hand- Calcium channels book). Specific applications of the ionic hypothesis to Equations of Ionic Hypothesis muscles, synapses, and sensory receptors are found in Solving Hodgkin-Huxley model Empirical constants other chapters in this Handbook. Solutions Significant features of membrane excitation can be seen by recording electric potential variations with The author’s original work was supported by grant NS 08174 an intracellular electrode. By convention the mem- from the National Institutes of Health. brane potential is defined as inside minus outside 99 100 HANDBOOK OF PHYSIOLOGY 8 THE NERVOUS SYSTEM I potential. The recorded potential suddenly becomes more negative as the recording electrode is moved from outside to inside, so excitable cells are said to 0 Frog have a negative resting potential. Again by conven- Node tion any potential change in the positive direction ,-25 22°C from rest is called a depolarization, whereas a change in the negative direction is called a hyperpolariza- tion. Intracellular records from several types of nerve -I75 d ‘L fiber and a motoneuron are shown in Figure 1. In 0 05 each example in Figure 1 excitation is initiated by a L..& m sec depolarizing electric shock, and the nerve cell re- sponds with a depolarizing electric response, the ac- tion potential, also called a spike or impulse. Action potentials are generally similar in all cells but differ in details of shape and time course from one cell type to another. The simple observation that both the stimulus and response are electric is intimately tied in with the mechanisrn of propagation of activity or conduction of action potentials. The response is said to be regenerative because electric currents generated by the action potential in one part of an axon are sufficient to excite the next part of the axon, and so 024 msec forth. Thus the impulse travels as a wave down the +so - length of an axon at fairly steady speed and ampli- tude. This voltage pulse is the unit of information in D nerve fibers and, as will be shown, is generated and >o n shaped by ionic permeability changes in each patch of E axon membrane. Sodium and potassium ions are by far the most important ions. Squid Axon 16°C DEVELOPMENT OF MEMBRANE THEORY - h Before Intracellular Recording 0 2 4 6 8 msec ORIGINS. The membrane theory dates from the begin- FIG. 1. Intracellularly recorded resting and action potentials ning of this century arid has roots in previous centu- from several nerve cells. A; single node of Ranvier of rat myelin- ries. At the end of the eighteenth century Galvani ated nerve fiber at 37°C. Brief stimulus applied at same node. (W. concluded that nerves and muscles use a flow of Nonner, M. Hordtkova, and R. Stampfli, unpublished data.) B: “animal electricity” to communicate with the brain. same type of recording from frog myelinated fiber as in A, but at He supposed that the body stores positive and nega- 22°C. [From Dodge (56).] C; cat lumbar spinal motoneuron at 3TC, excited antidromically by stimulation of motor axon. (W. E. tive electricity separated by insulators. In the nine- Crill, unpublished data.) I): propagating action potential in squid teenth century the electric currents of nerve and giant axon at 16°C. Stimulus applied about 2 cm from recording muscle were found to produce brief, externally nega- site. [From Baker et al. (23.) tive voltage pulses traveling at high velocity along nerves and muscles. Nerves were correctly likened to permeable to potassium ions at rest. Bernstein knew electrical cables with a surface insulation- the core that K+ is at higher concentration inside cells than conductor theory. There were arguments whether the outside. Thus K+ would tend to diffuse out, removing electricity is there all t.he time or is generated only at positive charge from the cell interior and setting up a the time of the impulse. Near the end of the century negative internal potential. The potential continues there was an essential advance in physical chemis- to develop until it is so large as to oppose the further try. Arrhenius (17) showed that salts dissociate into net emux of K+ (i.e., until diffusion forces and elec- ions. Then Nernst (180, 181) and Planck (191, 192) tric forces exactly cancel). For a membrane exclu- gave theories for equilibrium and diffusion potentials sively permeable to K+, the potential can be calcu- in ionic solutions, and Nernst and W. V. Ostwald lated from the Nernst equation for equilibrium poten- remarked that biological potentials might also be tials explainable by the same theories. The membrane theory is usually attributed to the physiologist Bernstein (26, 27) who suggested in 1902 that nerve and muscle cell membranes are selectively CHAF’TER 4: IONIC BASIS OF RESTING AND ACTION POTENTIALS 101 where [KJ, and [K], are the outside and inside potas- STRENGTH - DURATION sium ion concentrations, z the valence (+11, R the gas Toad sciatic nerve 10.2”C constant, T the absolute temperature, and F the Faraday. At 20°C the value of RTIF is 25.3 mV (see Table 4 in section EQUATIONS OF IONIC HYPOTHESIS). E is called the potassium equilibrium potential. For no concentration difference, E, is 0 mV, and for a 10- fold higher internal K+ concentration than outside, E, is -58.2 mV. Bernstein’s theory was suggested by I __---- the depolarizing effect of salts of potassium applied to I\-- f----- i- the surface of nerves. Bernstein (26) further sug- Rheobase gested that the propagated impulse involves “an in- 41 I f+ crease of membrane permeability for the retained ion 0 5 10 43 due to a chemical change of the plasma [membrane].” Shock duration (msec) In his view this wave of permeability change over- REFRACTORY PER I OD comes the potassium potential by allowing all ions to B, diffuse across the membrane simultaneously. In the 4-.f Frog sciatic nerve 148OC 2 (1 English-language literature the permeability in- t crease was dubbed a membrane “breakdown.” Unfor- ? 3- tunately intracellular recording was unknown in 1902, so most quantitative features of Bernstein’s proposal were temporarily untestable. STIMULATION. Bernstein’s ideas were generally ac- cepted for 40 years with little proof, as axonologists worked on questions approachable with extracellular recording. When a nerve is artificially stimulated by an externally applied electric shock, the impulse nor- I I 1 I mally arises at the electrode producing depolariza- 0 10 20 30 tion, the cathode or negative (external) electrode. Interval between shocks (msec) Large shocks stimulate more of the fibers in a nerve FIG. 2. Strength-duration curve and refractory period in large myelinated fibers of cooled amphibian sciatic nerve. Threshold than small shocks, and none of the fibers is excited if shock measured as the smallest amplitude shock to the nerve that the shock is too weak. Each individual fiber either makes a just-detectable twitch in an attached whole muscle. A: does or does not give a propagated response, depend- threshold shock amplitude vs. shock duration for a single rectan- ing on if the shock is greater or less than a critical gular shock. [Data from Lucas (163.1 B: threshold shock ampli- threshold value for that fiber.
Details
-
File Typepdf
-
Upload Time-
-
Content LanguagesEnglish
-
Upload UserAnonymous/Not logged-in
-
File Pages38 Page
-
File Size-