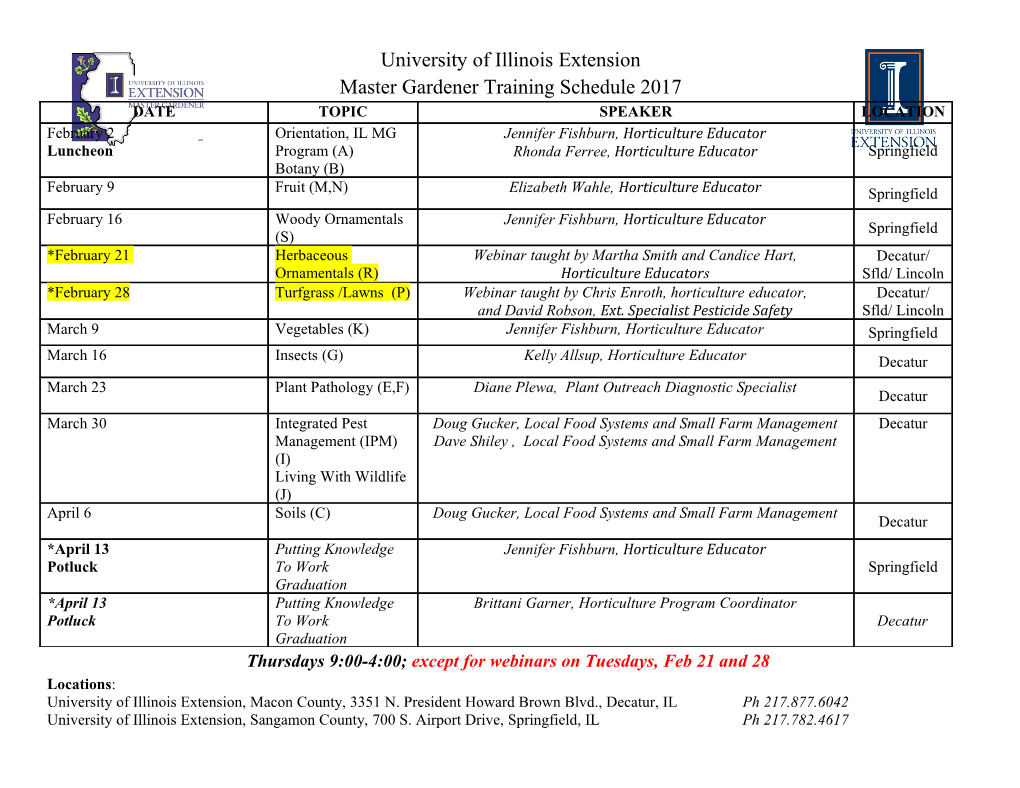
Application Note by QUANTELQUANTEL 1/7 Distribution: external EYLSA laser for atom cooling For decades, cold atom system and Bose-Einstein condensates (obtained from ultra-cold atoms) have been two of the most studied topics in fundamental physics. Several Nobel prizes have been awarded and hundreds of millions of dollars have been invested in this research. In 1975, cold atom research was enhanced through discoveries of laser cooling techniques by two groups: the first being David J. Wineland and Hans Georg Dehmelt and the second Theodor W. Hänsch and Arthur Leonard Schawlow. These techniques were first demonstrated by Wineland, Drullinger, and Walls in 1978 and shortly afterwards by Neuhauser, Hohenstatt, Toschek and Dehmelt. One conceptually simple form of Doppler cooling is referred to as optical molasses, since the dissipative optical force resembles the viscous drag on a body moving through molasses. Steven Chu, Claude Cohen-Tannoudji and William D. Phillips were awarded the 1997 Nobel Prize in Physics for their work in “laser cooling and trapping of neutral atoms”. In 2001, Wolfgang Ketterle, Eric Allin Cornell and Carl Wieman also received the Nobel Prize in Physics for realization of the first Bose-Einstein condensation. Also in 2012, Serge Haroche and David J. Wineland were awarded a Nobel prize for “ground-breaking experimental methods that enable measuring and manipulation of individual quantum systems”. Even though this award is more directed toward photons studies, it makes use of cold atoms (Rydberg atoms). Laser cooling Principle Sources: https://en.wikipedia.org/wiki/Laser_cooling and https://en.wikipedia.org/wiki/Doppler_cooling Laser cooling refers to a number of techniques in which atomic To a stationary atom the laser is neither red- and molecular samples are cooled to near absolute zero through nor blue-shifted and the atom does not absorb interaction with one or more laser fields. All laser cooling the photon. techniques rely on the property that when an object (usually an atom) absorbs and re-emits a photon (a particle of light) its To an atom moving away from the laser momentum changes. The temperature of a substance is higher the beam is red-shifted and the atom does not when there is a larger distribution of the velocities of the particles absorb the photon. that make up the substance. Laser cooling techniques combine To an atom moving towards the laser the beam atomic spectroscopy with the previously mentioned mechanical is blue-shifted (at its atomic transition wavelength) effect of light to compress the velocity distribution of a group of and the atom absorbs the photon, slowing it. particles, thereby cooling the particles. The absorbed photon excites the atom, The first example of laser cooling, and also the most common moving an electron to a higher quantum state. method (so much so that it is often simply referred to as 'laser cooling') is Doppler cooling (see Figure). The atom re-emits a photon, but the direction Doppler cooling uses a laser slightly red-shifted from the of the photon is random, so no net change molecule’s atomic transition with the result that: in momentum results over many absorption-emission cycles. Copyright © Quantel. All Rights Reserved. ANI001-version A. Quantel reserves the right to make changes without prior notice. A. ANI001-version All Rights Reserved. Copyright © Quantel. C i ht © Q t l All Ri ht R d AN001 i A Q t l th i ht t k h ith t i ti [email protected] Application Note by QUANTELQUANTEL 2/7 Distribution: Internalexternal only EYLSA laser for atom cooling Cooling atoms requires the photon absorption/emission cycle EYLSA fiber laser previously described to be reproduced a large number of times. Photons emitted by a laser source have the same direction. After a Wavelength coverage large number of absorption/emission cycles, the total force EYLSA fiber lasers are available at different wavelength covering: received by an atom resulting from absorption is equal to the sum • infrared (15XX and 10XX nm), of the force applied from each absorption multiplied by the number • near-IR (7XX nm), of absorptions. Whereas the total force applied to an atom • green (5XX nm) ranges. resulting from spontaneous emissions is quasi null (due to the random direction of photon emissions). Other wavelengths are currently under development. For this to happen, it is necessary that the atoms recover their (See graph 1 page 6) initial fundamental state through spontaneous emission in order to be excited again and so on. This is called cycling transition. Some Wavelength accuracy and stability atoms have a more complex structure, composed by 2 or more EYLSA wavelength accuracy may be specified at +/-0.01 nm using wavelength meters or atomic cells. energy levels within the atom (hyperfine structure), and may require the use of more than one cooling laser (see D2 transition of Frequency stability obtained from EYLSA 780 nm, wavelength locking the Rb structure). loop open. (see graph 2 page 6). F = 3 gF o=o2/3 Cooling (0.93 MHz/G) 193.7407(46) MHz 266.6500(90) MHz 2 5 P 3/2 72.9112(32)72.9 MHz F = 2 gF o=o2/3 229.8518(56) MHz (0.93 MHz/G) 302.0738(88)88) MHz 156.9470(70) MHz F = 1 gF o=o2/3 72.2180(40) MHz (0.93 MHz/G) F = 0 780.241 209 686(13) nm Repumping 384.230 484 468 5(62) THz 12 816.549 389 93(21) cm -1 1.589 049 462(38) eV F = 2 gF o=o1/2 (0.70 MHz/G) 2.563 005 979 089 109(34) GHz 2 5 S1/2 6.834.83 682 610 904 290(90) GHz 4.271 676 631 815 181(56) GHz F = 1 gF o=o-1/2 (-o0.70 MHz/G) Figure 1: D2 line of Rb87 and cycling transition Copyright © Quantel. All Rights Reserved. ANI001-version A. Quantel reserves the right to make changes without prior notice. A. ANI001-version All Rights Reserved. Copyright © Quantel. [email protected] Application Note by QUANTELQUANTEL 3/7 Distribution: external EYLSA laser for atom cooling Doppler cooling requires lasers that emit a very precise wavelength Wavelength locking that is precisely shifted from the target cycling transition (typically at EYLSA fiber lasers embed: 2.5Γ red shifted for optimal atom cooling where Γ is the natural • a monitoring fiber output that may be directly connected to a linewidth of the transition; the shift height is determined by the initial saturated absorption scheme (standard cold atom lab apparatus, atom speeds). The specified wavelength accuracy must be better not provided with EYLSA) . than the transition linewidth (~MHz). Most of the time, a wavelength • a wavelength locking input (BNC) which directly modifies seeder locking system is required to reference the laser to the atomic diode power supply current. Wavelength is tunable on a transition during long time periods. Also, laser linewidth must be reproducible and mode-hop-free (MHF) range. This input may be lower than the atomic transition for better photon efficiency and to connected to saturated absorption equipment associated with PID avoid excitation of other atomic transitions with out-of-band (not included with EYLSA) to lock the EYLSA fiber laser wavelength. photons. Atoms excited at another transition may become insensitive to the apparatus and won’t be cooled and may even Successful long-term locking has been demonstrated without relocking: warm other atoms by collisions. For this same reason the laser’s • in lab conditions for longer than 2 weeks optical signal to noise ratio must be excellent. (This requires there • in high vibration and temperature fluctuation conditions such as are no parasitic laser lines (mode-hop) and very limited amplified the 0G flight ICE experiment, Bordeaux (20 parabolas) spontaneous emission). Fully available output power Note: For atom interferometry experiments (precision Each photon generated from an EYLSA product is fully available for measurement techniques using cold atoms which have been the experiment: developed in many labs), laser linewidth is required to be narrower • No need for mode filtering by few orders, for example kHz to Hz linewidth may be necessary. • No need for fiber injection (the photon is already injected into the fiber output) In practice, an atomic cloud of mK temperature is obtained by • No need to push the current up to keep a constant output power trapping the atoms in a vacuum chamber and combining the laser over the lifecycle. cooling principle oriented in 3 directions and a magnetic field. The Optical signal to noise ratio magnetic field is designed to be 0 at the experiment chamber EYLSA fiber lasers generate visible light from IR light using center and increase proportionally to the distance from the periodically-poled crystals. chamber center. The purpose of the magnetic field is to slightly • Second-harmonic generation is a non-linear effect compared to shift the atom’s hyperfine structures. Thus, lasers slightly shifted incident power. The higher the incident power the higher the from the transition wavelength associated with this magnetic field conversion efficiency is. will exert a restoring force proportional to the atom’s distance • Periodically-poled crystals have a limited spectral acceptance from the center of the chamber. This type of trap is called 3D range of 20 GHz, any photon outside this range is filtered out. Magneto-Optical Trap (3D MOT). Optical signal to noise ratio (OSNR) is measured at better than 55 dB For this trap, 6 beams are required to trap the atoms in each spatial for a standard frequency double laser. (see graph 3 page 6). direction and within the 2 propagating paths.
Details
-
File Typepdf
-
Upload Time-
-
Content LanguagesEnglish
-
Upload UserAnonymous/Not logged-in
-
File Pages7 Page
-
File Size-