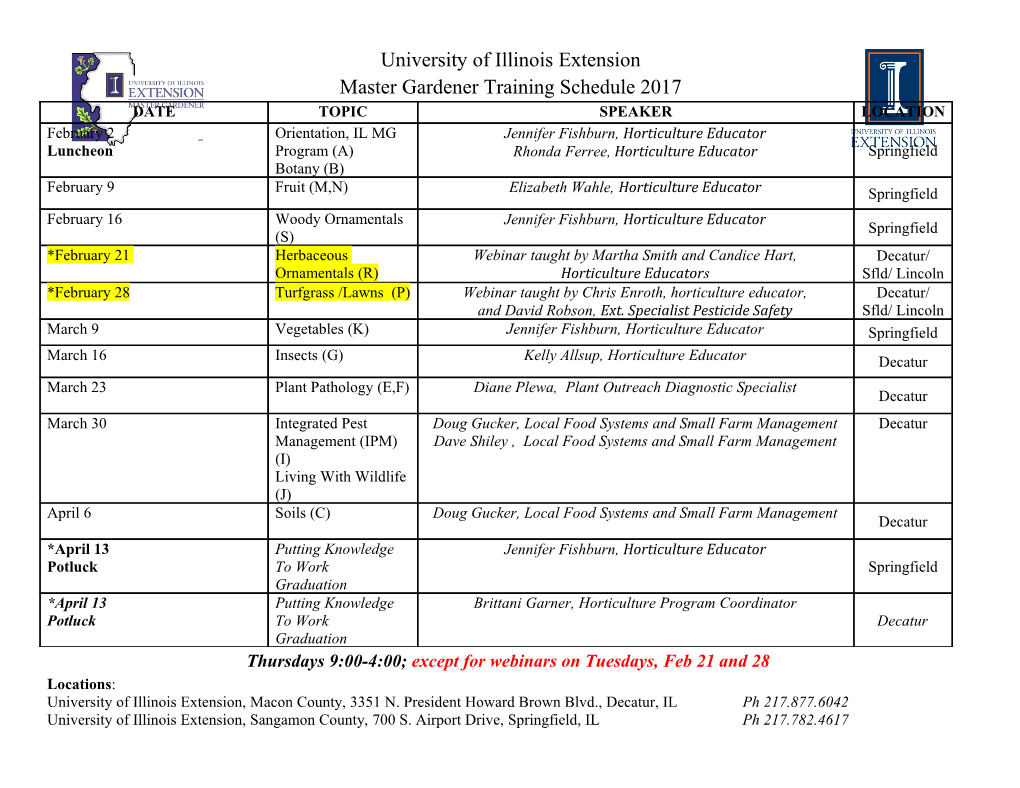
Progress in Materials Science 94 (2018) 68–113 Contents lists available at ScienceDirect Progress in Materials Science journal homepage: www.elsevier.com/locate/pmatsci Bespoke photonic devices using ultrafast laser driven ion migration in glasses ⇑ T.T. Fernandez a,1, M. Sakakura b,1, S.M. Eaton a, B. Sotillo a, , J. Siegel c, J. Solis c, Y. Shimotsuma d, K. Miura d a Istituto di Fotonica e Nanotecnologie-Consiglio Nazionale delle Ricerche (IFN-CNR), Milano, Italy b Optoelectronics Research Centre, University of Southampton, Southampton, United Kingdom c Laser Processing Group, Instituto de Optica, Consejo Superior de Investigaciones Científicas (IO,CSIC), Madrid, Spain d Department of Materials Chemistry, Kyoto University, Kyoto, Japan article info abstract Article history: This Review provides an exhaustive and detailed description of ion migration phenomena Received 16 August 2016 which occur inside transparent dielectric media due to the interaction with intense ultra- Accepted 20 December 2017 short pulses. The paper differentiates various processes underlying the ion migration influ- Available online 29 December 2017 enced by simultaneous heat accumulation and diffusion. The femtosecond laser induced temperature distribution, the major driving force of ions in dielectrics, is described in detail. Keywords: This discussion is based on three meticulous analysis methods including the thermal modi- Femtosecond laser micromachining fication of transparent dielectrics at various ambient temperatures, numerical simulations Ion-migration and comparison with direct observation of the light-matter interaction and micro-Raman Scanning electron microscope Glass spectroscopy. The ion migration phenomena studied have been triggered in four different Waveguides configurations: at low repetition and high repetition rates, and observations perpendicular Photonic devices and parallel to the laser irradiation direction. Inspired by this research, potential applications are highlighted including space-selective phase separation, a laser-based ion exchange fabrication method and optical micropipetting by tailoring the plasma profile. Ó 2018 Elsevier Ltd. All rights reserved. Contents 1. Introduction . ................................................................................ 70 2. Background. ................................................................................ 70 2.1. Femtosecond laser sources . ...................................................................... 70 2.2. Femtosecond laser microfabrication . ................................................... 71 2.2.1. Nonlinear absorption . ........................................................ 71 2.2.2. Relaxation and material modification . ........................................................ 72 2.3. Dielectric materials used for fabrication . ................................................... 74 2.4. Characterization techniques . ...................................................................... 74 3. Basic principle of heat accumulation and ion migration by fs laser irradiation. .......................... 75 3.1. Observation of thermal diffusion after single fs laser irradiation inside a glass . ................................ 77 3.1.1. Observation of thermal diffusion by a transient lens (TrL) method . .................. 77 ⇑ Corresponding author at: Istituto di Fotonica e Nanotecnologie-Consiglio Nazionale delle Ricerche(IFN-CNR), Milano, Italy (B. Sotillo). E-mail address: [email protected] (B. Sotillo). 1 Co-first authors. https://doi.org/10.1016/j.pmatsci.2017.12.002 0079-6425/Ó 2018 Elsevier Ltd. All rights reserved. T.T. Fernandez et al. / Progress in Materials Science 94 (2018) 68–113 69 Nomenclature Acronym Meaning AR Aspect Ratio Bi Bidirectional pumping BSE Back Scattered Electrons CCD Charge-Coupled Device CW Continuous-Wave DIC Differential Interference Contrast EBSD Electron Back Scattered Diffraction EDS, EDX Energy Dispersive X-ray Spectroscopy EELS Electron Energy Loss Spectroscopy EPMA Electron Probe Microanalysis FT Fourier Transform HAADF High Angle Annular Dark Field HRR High Repetition Rate ICCD Intensified Charge-Coupled Device IFT Iterative Fourier transform IG Internal Gain LCOS Liquid Crystal on Silicon LRR Low Repetition Rate NA Numerical Aperture OM Optical Microscope ORA Optimal Rotation Angle ppmw Parts Per Million Weight R Repetition rate RE Rare-Earth RIC Refractive Index Contrast SA Spherical Aberration SEM Scanning Electron Microscopy SLM Spatial Light Modulator STEM Scanning Transmission Electron Microscopy Tout Threshold Temperature TEM Transmission Electron Microscopy TL Thermal Lens TrL transient Lens ULE Ultra-Low Expansion Uni Unidirectional pumping WDS Wavelength Dispersive Spectroscopy m-PL Micro-Photoluminescence m-Raman Micro-Raman 3.1.2. Observation of thermal diffusion by micro Raman measurement. ............................ 79 3.2. Heat accumulation with a high repetition rate laser . .......................................... 80 3.2.1. Simulation of heat accumulation. ............................................... 80 3.2.2. Evaluation of the temperature distribution during high repetition rate laser irradiation . ......... 81 3.2.3. Mechanism of thermal modification inside glasses . ............................................... 84 3.2.4. Interpretation of different Tout by the viscoelastic model . ............................ 86 3.3. Absorptivity and photoexcitation mechanism............................................................. 87 3.4. Trends of ion migration observed perpendicular to the incident laser . ....................... 89 4. High repetition rate laser irradiation . ....................................................................... 91 4.1. Observation of ion migration with high repetition rate lasers . .......................................... 91 4.1.1. Ion migration in phosphate glass waveguides . ............................................... 94 4.1.2. Ion migration in tellurite glass waveguides . ............................................... 94 4.1.3. Dual regimes of ion migration .................................................................. 95 4.2. Controlling the directionality of ion migrations . .......................................... 97 5. Application of ion migration . ...................................................................... 100 5.1. Space-selective phase separation . ............................................................ 100 5.2. Replacing the ion exchange method . ............................................................ 101 5.3. Optical micropipette through plasma shaping . ......................................... 103 6. Modification of ion migration with multiple spot irradiation . ................................................... 103 6.1. Modification of ion migration. ............................................................ 103 70 T.T. Fernandez et al. / Progress in Materials Science 94 (2018) 68–113 6.2. Principle of a holographic laser irradiation . .................................................. 104 6.3. Modification of ion migration perpendicular to the laser propagation . ............................... 105 6.4. Modification of ion migration parallel to the laser propagation . ............................... 106 6.5. Possible applications of ion migration with multiple spot irradiation. ............................... 109 7. Conclusion . ............................................................................... 109 References . ............................................................................... 109 1. Introduction Studying the underlying physical mechanisms from its smallest possible dimension provides extraordinary precision and control for any experimental technique. One such method that has benefited from new physical insights is ultrafast laser waveguide writing in glasses. Here, femtosecond laser pulses are focused beneath the surface of glass, with light nonlinearly absorbed to yield a permanent and localized refractive index modification. By translating the sample relative to the laser, optical waveguides can be inscribed along 3D trajectories, enabling novel integrated optical devices ranging from UV [1] to Terahertz [2]. However, controlling the refractive index modification, which affects the quality of photonic circuits, is a challenge that has yet to be met by researchers. Since the pioneering work by Miura’s group in 1996, bulk modification of glasses by ultrafast laser irradiation has been studied extensively, with over two thousand papers citing the seminal paper [3]. Several mechanisms have been proposed to explain the ultrafast laser induced refractive index change, including heat accumulation [4,5], thermal quenching [6,7], structural modification [8], color center formation [9], shock wave propagation and nanograting formation [10]. All of these effects influence the resulting morphological change, but their prominence depends on the laser processing conditions and glass type. The crucial role of ion migration during femtosecond laser writing has been disregarded until only recently. The first ever report of ion migration was from Miura’s group while studying space selective crystallization in amorphous dielec- trics using a 800 nm, 130 fs, 200 kHz femtosecond laser [11]. The authors suggested that crystal nuclei are formed at points where the crystallization temperature is exceeded, due to atomic diffusion and the microstructure rearrangement. As described in this Review, ion migration
Details
-
File Typepdf
-
Upload Time-
-
Content LanguagesEnglish
-
Upload UserAnonymous/Not logged-in
-
File Pages46 Page
-
File Size-