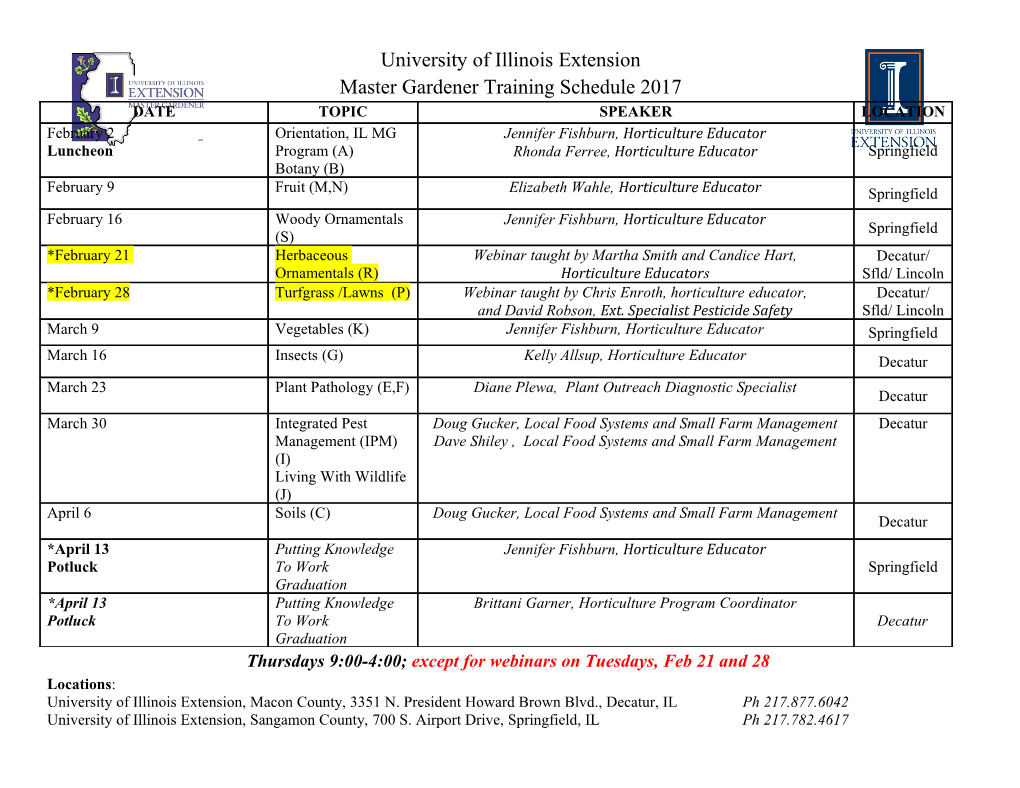
1 2 3 4 Article type : Special Feature Review 5 6 7 8 9 Surveying purine biosynthesis across the domains of life 10 unveils promising drug targets in pathogens 11 12 13 14 Sheena M. H. Chua and James A. Fraser* 15 16 17 18 Australian Infectious Diseases Research Centre 19 School of Chemistry & Molecular Biosciences 20 The University of Queensland, Brisbane, Queensland, Australia 21 22 23 24 25 26 27 28 29 30 Manuscript Author 31 *Correspondence e-mail: [email protected] This is the author manuscript accepted for publication and has undergone full peer review but has not been through the copyediting, typesetting, pagination and proofreading process, which may lead to differences between this version and the Version of Record. Please cite this article as doi: 10.1111/IMCB.12389 This article is protected by copyright. All rights reserved 32 Running head 33 34 Purine biosynthesis across the domains of life 35 36 37 Keywords 38 39 Drug target, gene fusions, immunotherapy, infectious diseases, purine metabolism, 40 purinosome 41 42 Abstract 43 Purines play an integral role in cellular processes such as energy metabolism, cell signalling, 44 and encoding the genetic makeup of all living organisms, ensuring that the purine metabolic 45 pathway is maintained across all domains of life. To gain a deeper understanding of purine 46 biosynthesis via the de novo biosynthetic pathway, the genes encoding purine metabolic 47 enzymes from 35 archaea, 69 bacteria and 99 eukaryotic species were investigated. While the 48 classic elements of the canonical purine metabolic pathway were utilised in all domains, a 49 subset of familiar biochemical roles were found to be performed by unrelated proteins in 50 some members of the Archaea and Bacteria. In the Bacteria, a major differentiating feature of 51 de novo purine biosynthesis is the increasing prevalence of gene fusions, where two or more 52 purine biosynthesis enzymes that perform consecutive biochemical functions in the pathway 53 are encoded by a single gene. All species in the Eukaryota exhibited the most common 54 fusions seen in the Bacteria, in addition to new gene fusions to potentially increase metabolic 55 flux. This complexity is taken further in humans, where a reversible biomolecular assembly 56 of enzymes known as the purinosome has been identified, allowing short-term regulation in 57 response to metabolic cues whilst expanding on the benefits that can come from gene fusion. 58 By surveying purine metabolism across all domains of life we have identified important 59 features of the purine biosynthetic pathway that can potentially be exploited as prospective 60 drug targets. Author Manuscript Author 61 62 Purines and the origin of life This article is protected by copyright. All rights reserved 63 The most widely accepted model for the origin of life is that it arose via creation of organic 64 molecules produced by simple prebiotic chemical reactions. The Miller-Urey experiment 65 showed that amino acids essential for creating proteins could be formed from simple 66 molecules like water, methane, ammonia and hydrogen.1 Further investigations established 67 that the purine nucleotides adenine and guanine, key components of both RNA and DNA, 68 could also form under conditions likely present on primitive Earth.2 Over 3.5 billion years 69 later, all living things from the simplest unicellular life to more complex multicellular 70 organisms require purines for survival. Purines play an integral role in diverse processes 71 including energy metabolism, cell signalling, and encoding the genetic makeup of all 72 organisms – providing strong selective pressure that ensures the purine de novo biosynthetic 73 pathway is maintained across all domains of life. 74 75 Purines contain a six-membered pyrimidine ring fused to a five-membered imidazole ring. 76 The first of these heterocyclic compounds were discovered in urinary calculi; uric acid (from 77 the French urique for “urine”) in 1776 and xanthine (from the Greek xanthos for “yellow”) in 78 1838.3, 4 Guanine was identified in 1846 and named for the bird guano in which it was found, 79 and four years later hypoxanthine (“xanthine with less oxygen”) was isolated from cow 80 spleen.5, 6 It was not until 1884 that the term “purine” was coined from the Latin purum 81 (“pure”) and urinae (“urine”) by Fischer, who was awarded a Nobel Prize for his 82 achievements in the field of purine synthesis.7 Experiments on ox pancreas in 1886 led to the 83 identification of adenine (from the Greek aden for “gland”) that in turn led to Kossel being 84 awarded a Nobel Prize for the discovery that nucleic acids were composed of the purines 85 adenine and guanine alongside the pyrimidines thymine, cytosine and uracil.8 86 87 The biochemical processes underpinning the synthesis of purines would not be identified 88 until over half a century later. The determination of the intermediates involved in de novo 89 purine biosynthesis began in the 1930s with the discovery that hypoxanthine could be 90 detected in the livers of pigeons but not chickens, ducks, rats or guinea pigs.9 Key 91 intermediates in the pathway were subsequently elucidated by feeding isotopically labelled 92 substrates to pigeons and analysing uric acid crystallised from their droppings.10 These in Author Manuscript Author 93 vivo experiments were followed by in vitro studies purifying and assaying individual 94 enzymes from pigeon liver, cow liver, and Saccharomyces cerevisiae, together laying the 95 foundation for our current understanding of the biochemical processes required for the de 96 novo biosynthesis of purines in the Eukaryota.11-13 This article is protected by copyright. All rights reserved 97 98 The biochemistry of purine biosynthesis in the Eukaryota 99 De novo purine and pyrimidine biosynthesis both require the ATP-dependent 100 phosphorylation of ribose-5-phosphate (R5P) by ribose-phosphate diphosphokinase (EC 101 2.7.6.1) to produce phosphoribosyl-pyrophosphate (PRPP).14 102 103 The first dedicated step of de novo purine biosynthesis is the hydrolysis of L-glutamine and 104 transfer of the liberated amine group to PRPP by PRPP amidotransferase (PRPPA, EC 105 2.4.2.14), creating phosphoribosyl-amine (PRA; Figure 1).15 Next, phosphoribosyl- 106 glycinamide (GAR) synthetase (GARS, EC 6.3.4.13) catalyses the ATP-dependent ligation of 107 L-glycine to PRA (via a phosphorylated intermediate) to yield GAR.16 GAR transformylase 108 (N10-fTHF-GART, EC 2.1.2.2) then ligates the formyl group from 10-formyltetrahydrofolate 109 (N10-fTHF) to GAR, producing phosphoribosyl-formylglycinamide (FGAR).17 The 110 subsequent activation of the FGAR amide oxygen by the ATP-grasp domain of 111 phosphoribosyl-formylglycinamidine (FGAM) synthetase (FGAMS, EC 6.3.5.3) produces an 112 iminophosphate intermediate that is amidated by ammonia, channelled via a structural 113 domain, from the glutaminase domain to create FGAM.18 Finally, phosphoribosyl- 114 aminoimidazole (AIR) synthetase (AIRS, EC 6.3.3.1) catalyses the ATP-dependent activation 115 of the FGAM formyl oxygen that reacts with a nearby nitrogen to close the five-membered 116 imidazole ring of the purine base and form AIR.19 117 118 Formation of the pyrimidine ring then begins with phosphoribosyl-carboxyaminoimidazole 119 (CAIR) synthetase (CAIRM-II type CAIRS, EC 4.1.1.21) carboxylating AIR using CO2 to 120 form CAIR.20 Phosphoribosyl-aminoimidazolesuccinocarboxamide (SAICAR) synthetase 121 (SAICARS, EC 6.3.2.6) then mediates the ATP-dependent ligation of L-aspartate to CAIR 122 forming SAICAR; the following -elimination of fumarate from SAICAR by 123 adenylosuccinate (ADS) lyase (ADSL, EC 4.3.2.2) produces phosphoribosyl- 124 aminoimidazolecarboxamide (AICAR).21 AICAR transformylase (N10-fTHF-AICART, EC 125 2.1.2.3) then ligates the formyl group from N10-fTHF to AICAR to form phosphoribosyl- 22 126 formamidocarboxamide Manuscript Author (FAICAR). Closure of the pyrimidine ring (completing formation 127 of the purine base) occurs via elimination of water from FAICAR by inosine monophosphate 128 (IMP) cyclohydrolase (IMPC, EC 3.5.4.10) and cyclisation to produce IMP.23 129 This article is protected by copyright. All rights reserved 130 Purine de novo biosynthesis bifurcates after IMP. In the adenosine monophosphate (AMP) 131 biosynthesis branch, ADS synthetase (ADSS, EC 6.3.4.4) transfers the γ-phosphate from GTP 132 to IMP, forming the intermediate 6-phosphoryl IMP; the 6-phosphoryl group is then 133 displaced by the α-amino group of L-aspartate to form ADS.24, 25 The conversion of ADS to 134 AMP follows the same -elimination mechanism by ADS lyase that it performs earlier in the 135 pathway.26, 27 AMP can be converted to ADP by adenylate kinase (ADK), then ATP by 136 nucleoside diphosphate kinase (NDK).28, 29 In the GMP biosynthesis branch, IMP 137 dehydrogenase (IMPDH, EC 1.1.1.205) catalyses the NAD+-dependent hydrolysis of IMP to 138 form XMP.30, 31 Next, the ATP pyrophophatase domain of GMP synthetase (GMPs, EC 139 6.3.5.2) adenylates XMP to form an XMP intermediate, while the glutamine amidotransferase 140 domain produces ammonia to add an amine group to the intermediate and yield GMP.27 GMP 141 can be converted to GDP by guanylate kinase (GUK), then GTP by nucleoside diphosphate 142 kinase (NDK).29, 32 143 144 In addition to the de novo pathway, exogenous purines can also be scavenged from the 145 environment via a salvage pathway through the action of phosphoribosyltransferases that 146 convert adenine, hypoxanthine, xanthine and guanine to AMP, IMP, XMP and GMP, 147 respectively.31, 33 148 149 The history of purine metabolism as a drug target 150 Given the requirement for significant quantities of nucleic acids in highly proliferative cells 151 such as cancers, immune cells and infecting pathogens, it was proposed that targeting of 152 specific biological processes in the purine metabolism pathway may lead to effective 153 therapeutic treatments.34, 35 This premise led to the conception of rational drug design, 154 earning Hitchings and Elion a Nobel Prize.36 155 156 Pharmaceuticals that act through the purine biosynthetic pathway were subsequently 157 developed.
Details
-
File Typepdf
-
Upload Time-
-
Content LanguagesEnglish
-
Upload UserAnonymous/Not logged-in
-
File Pages26 Page
-
File Size-