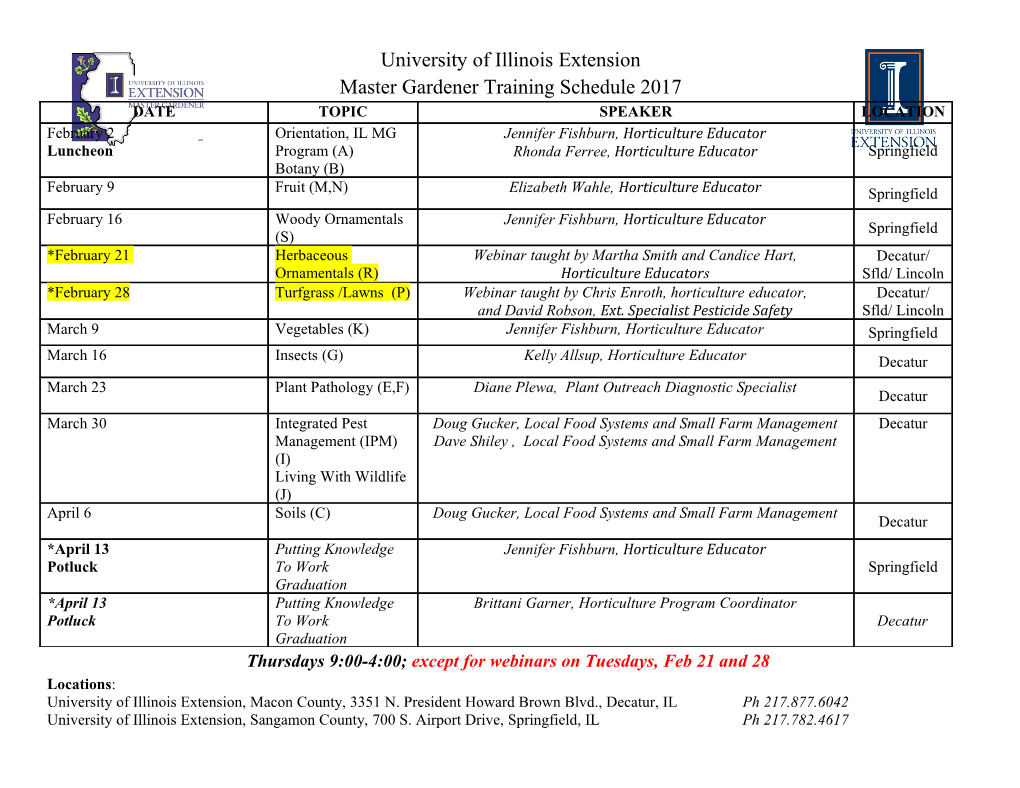
13. Weathering Processes By W. Ian Ridley 13 of 21 Volcanogenic Massive Sulfide Occurrence Model Scientific Investigations Report 2010–5070–C U.S. Department of the Interior U.S. Geological Survey U.S. Department of the Interior KEN SALAZAR, Secretary U.S. Geological Survey Marcia K. McNutt, Director U.S. Geological Survey, Reston, Virginia: 2012 For more information on the USGS—the Federal source for science about the Earth, its natural and living resources, natural hazards, and the environment, visit http://www.usgs.gov or call 1–888–ASK–USGS. For an overview of USGS information products, including maps, imagery, and publications, visit http://www.usgs.gov/pubprod To order this and other USGS information products, visit http://store.usgs.gov Any use of trade, product, or firm names is for descriptive purposes only and does not imply endorsement by the U.S. Government. Although this report is in the public domain, permission must be secured from the individual copyright owners to reproduce any copyrighted materials contained within this report. Suggested citation: Ridley, W. Ian, 2012, Weathering processes in volcanogenic massive sulfide occurrence model: U.S. Geological Survey Scientific Investigations Report 2010–5070 –C, chap. 13, 8 p. 193 Contents Mineralogic Reactions to Establish Process ........................................................................................195 Geochemical Processes ...........................................................................................................................196 Factors Controlling Rates of Reaction ....................................................................................................198 Effects of Microclimate and Macroclimate ..........................................................................................199 Effects of Hydrologic Setting ...................................................................................................................199 References Cited........................................................................................................................................200 13. Weathering Processes By W. Ian Ridley Seafloor massive sulfides (SMS), the presumed precur- Mineralogic Reactions to Establish sors of ancient VMS deposits, are unstable in the presence of even small concentrations of oxygen. They are potentially sub- Process jected to ambient temperature weathering and other processes that can cause substantial postdepositional changes in mineral- The physical and chemical degradation of a deposit is ogy and geochemistry (Koski and others, 2003). Submarine largely determined by the rates of oxidation of sulfides. There weathering (halmyrolysis) at the site of ore deposition results is a substantive amount of literature on the mechanisms and from a variety of reactions that are initially mediated by oxy- rates of pyrite oxidation (see Evangelou, 1995, for a review) genated seawater that circulates through the deposit. Oxidation but less information on other sulfides that are commonly found begins at the deposit/seawater interface, and the oxidation in VMS deposits. Pyrite can be directly oxidized by seawater front gradually moves into the deposit, resulting in mass wast- oxygen depending upon the oxygen concentration, which is a ing of the ore deposit at ambient temperatures of 1−2 °C. function of the rate of fresh seawater renewal and the degree In principle, the process of seafloor weathering can of bottom water anoxia: produce intermediate stages of supergene enrichments by 2+ 2- FeS2(s) + 7/2O2(aq) + H2O(l) → Fe (aq) + 2SO4 (aq) complete or partial replacement of primary sulfides by second- + ary sulfides and enriched gossans (Hannington and others, + 2H (aq) (1) and 1988; Herzig and others, 1991), but the continued circulation 4FeS (s) + 14O (aq) + 4H O(l) → 4FeSO (aq) + of oxygenated seawater eventually results in the physical and 2 2 2 4 4H SO (aq) (2) chemical destruction of the deposit. Consequently, weather- 2 4 ing of SMS deposits is an inevitable consequence of exposure Both reactions produce significant acidity, but the extent to seawater unless the deposit is rapidly covered by sediment to which the fluid acidity is maintained is determined by and(or) volcanic material that shields sulfides from seawater the degree to which these initial reactions occur in a closed contact. Anoxic, circumneutral bottom waters also contain a system. Pyrite oxidation also can be accomplished through small amount of oxygen so that, under anoxic conditions, the indirect exothermic reactions using oxygen and ferric iron. weathering of the deposit may be slowed but not halted. Solubility of Fe(III) is strongly pH dependent; however, even Microbial degradation of seafloor sulfides—for instance, in circumneutral seawater the concentrations of Fe(III) are suf- pyrrhotite—also may be an important process of seafloor ficient to dominate the oxidation of iron sulfides. In a system weathering (Toner and others, 2009). If a VMS deposit is that is open to seawater circulation, the acidity and dissolved preserved in the rock record, which must be a relatively rare iron are removed into the large reservoir of ocean water. The event, it may be subject to subaerial degradation if exposed to presence of a carapace of seafloor gossan, composed prin- abiotic reactions involving surface and near-surface oxygen- cipally of ferric oxides and oxy-hydroxides, reflects the low ated fluids, atmospheric oxygen, and biotic reactions involving solubility of Fe(III) in solution and implies fluid pH values bacterial activity. Halmyrolysis and subaerial degradation that are either neutral or slightly alkaline: require similar oxidative chemical reactions, the differences being largely determined by the presence or absence of gas- 2+ + 3+ Fe (aq) + 1/4O2(aq) + H (aq) → Fe (aq) + 1/2H2O(l) (3) eous oxygen, dissolved oxygen and iron concentrations, and and hydrologic setting. The deleterious environmental conse- 3+ + Fe (aq) + 3H20(l) → Fe(OH)3 +3H (4) quences of subaerial degradation of VMS ores and the result- ing acid drainage production, has received particular attention These reactions continue until the solid pyrite is from environmental geochemists over the past few decades exhausted or the sulfidic material is isolated from ambi- (Evangelou, 1995; Plumlee, 1999). ent seawater. The presence of a surface in situ gossan may 196 Chapter 13. Weathering Processes substantially slow down the rate at which the underlying Bornite: sulfidic material is oxidized. Initially, gossans have a high Cu3FeS(s) + 31/4O2(aq) + 7/2H2O(l) → Fe(OH)3(s) + 2- 2+ + porosity and permeability, so they do not form an efficient 4SO4 (aq) + 3Cu (aq) +4H (aq) (7) impermeable barrier to the circulation of seawater, but, upon Arsenopyrite: compaction, gossans can form a highly effective impermeable FeAsS(s) + 7/2O2(aq) +3H2O(l) → FeAsO4.2H20(s) 2- + barrier. The slowest step of reactions 1-4, and possibly other [scorodite] +SO4 (aq) + 2H (aq) (8) intermediate steps, initially determines the rate of pyrite oxida- Fe-rich sphalerite: tion. However, the rate of oxidation is also a function of avail- (Zn,Fe)S(s) + 3O2(aq) + H2O(l) → Fe(OH)3(s) + 2- 2+ + able dissolved oxygen and the extent to which ferric-bearing SO4 (aq) + Zn (aq) +2H (aq) (9) minerals encapsulate, and hence shield, pyrite from reaction In these cases, the reduced dissolved metal species are with oxygenated seawater. more soluble than dissolved Fe(III) and(or) their precipitation The origin of gossans associated with VMS deposits is is kinetically inhibited, so that Fe(III)-bearing minerals tend to not clearly established. Some appear to have formed on the dominate the mineralogy of seafloor gossans associated with seafloor whilst others are related to subaerial oxidation. The VMS deposits. Initially, however, the presence of cations other chemistry of seafloor gossans (Mills and others, 2001) has than iron in solution results in replacement of iron sulfides by been interpreted in terms of precipitation from low-tempera- secondary minerals, such as copper, zinc and lead sulfides, and ture vent fluids and hydrogenous reactions involving ambient possibly sulfates. The different solubilities of ferric iron and seawater. However, seafloor weathering of unstable sulfide manganese can result in large variations in the Fe/Mn ratios of minerals ultimately produces metal oxides and oxyhydroxides, seafloor gossans (Mills and others, 2001). Common minerals so some gossans must be a product of halmyrolysis. It seems formed during halmyrolysis include goethite, hematite, birnes- likely that seafloor gossans represent a spectrum of overlap- site, todorike, Fe-smectite, and various silica polymorphs. Iron ping processes involving low-temperature vent fluids and silicates, usually smectite, can also occur as a major compo- ambient seawater. nent of several “postdepositional” hydrothermal deposits. Modern SMS deposits are the sites of abundant micro- Koski and others (2008) determined a resistance to and macrofauna, and fossilized macrofauna have been encoun- oxidation in the subaerial environment in the order pyrrho- tered in ancient VMS deposits, such as those in the southern tite < sphalerite < chalcopyrite < pyrite, but it is unknown if Urals (Little and others, 1997) and the Troodos (Little and oth- this sequence also applies to the seafloor environment. The ers, 1999). Because the food chain for macro fauna begins at common presence of sphalerite in seafloor gossans suggests the bacterial level,
Details
-
File Typepdf
-
Upload Time-
-
Content LanguagesEnglish
-
Upload UserAnonymous/Not logged-in
-
File Pages11 Page
-
File Size-