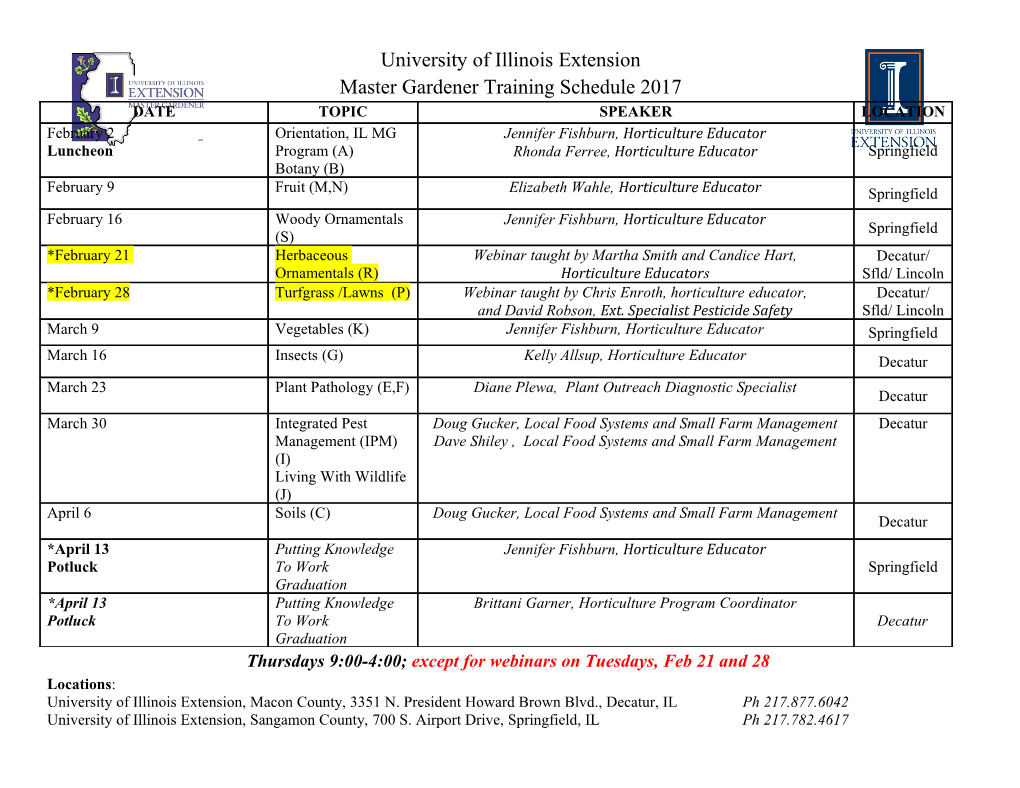
A&A 631, A103 (2019) Astronomy https://doi.org/10.1051/0004-6361/201935710 & © ESO 2019 Astrophysics Linking the evolution of terrestrial interiors and an early outgassed atmosphere to astrophysical observations Dan J. Bower1, Daniel Kitzmann1, Aaron S. Wolf2, Patrick Sanan3, Caroline Dorn4, and Apurva V. Oza5 1 Center for Space and Habitability, University of Bern, Gesellschaftsstrasse 6, 3012 Bern, Switzerland e-mail: [email protected]; [email protected] 2 Earth and Environmental Sciences, University of Michigan, 1100 North University Avenue, Ann Arbor, MI 48109-1005, USA e-mail: [email protected] 3 Institute of Geophysics, ETH Zurich, Sonneggstrasse 5, 8092 Zurich, Switzerland 4 University of Zurich, Institute of Computational Sciences, Winterthurerstrasse 190, 8057 Zurich, Switzerland 5 Physics Institute, University of Bern, Sidlerstrasse 5, 3012 Bern, Switzerland Received 16 April 2019 / Accepted 6 September 2019 ABSTRACT Context. A terrestrial planet is molten during formation and may remain molten due to intense insolation or tidal forces. Observations favour the detection and characterisation of hot planets, potentially with large outgassed atmospheres. Aims. We aim to determine the radius of hot Earth-like planets with large outgassing atmospheres. Our goal is to explore the differ- ences between molten and solid silicate planets on the mass–radius relationship and transmission and emission spectra. Methods. An interior–atmosphere model was combined with static structure calculations to track the evolving radius of a hot rocky planet that outgasses CO2 and H2O. We generated synthetic emission and transmission spectra for CO2 and H2O dominated atmo- spheres. Results. Atmospheres dominated by CO2 suppress the outgassing of H2O to a greater extent than previously realised since previ- ous studies applied an erroneous relationship between volatile mass and partial pressure. We therefore predict that more H2O can be retained by the interior during the later stages of magma ocean crystallisation. Formation of a surface lid can tie the outgassing of H2O to the efficiency of heat transport through the lid, rather than the radiative timescale of the atmosphere. Contraction of the mantle, as it cools from molten to solid, reduces the radius by around 5%, which can partly be offset by the addition of a relatively light species (e.g. H2O versus CO2) to the atmosphere. Conclusions. A molten silicate mantle can increase the radius of a terrestrial planet by around 5% compared to its solid counterpart, or equivalently account for a 13% decrease in bulk density. An outgassing atmosphere can perturb the total radius, according to its composition, notably the abundance of light versus heavy volatile species. Atmospheres of terrestrial planets around M-stars that are dominated by CO2 or H2O can be distinguished by observing facilities with extended wavelength coverage (e.g. JWST). Key words. planets and satellites: interiors – planets and satellites: detection – planets and satellites: terrestrial planets – Earth – planets and satellites: atmospheres – planets and satellites: composition 1. Introduction The earliest secondary atmosphere of a rocky planet origi- nates from extensive volatile release during one or more magma The detection of over 1000 rocky exoplanets has ushered in a ocean epochs that occur during and after the assembly of the new era of exoplanet characterisation, which is driven by the goal planet. Magma oceans form as a result of accretion, core- of identifying and characterising Earth-analogues or potentially formation, radioactive decay of short-lived elements, and giant habitable worlds. During planet formation, terrestrial planets do impacts (e.g. Elkins-Tanton 2012). For example, the moon- not retain a significant primordial atmosphere from the neb- forming impact melted at least two-thirds of Earth’s mantle ula gas due to volatile loss during accretion (e.g. Schlichting & (Nakajima & Stevenson 2015), producing a global magma ocean Mukhopadhyay 2018). Rather, they outgas volatiles from the that subsequently cooled and crystallised. Magma oceans set the interior to form so-called secondary atmospheres once the cir- stage for the long-term evolution of terrestrial planets by estab- cumstellar disk decays. This strong coupling between the rocky lishing the major chemical reservoirs of the iron core and silicate interior and atmosphere presents an opportunity to probe the mantle, chemical stratification within the mantle, and outgassed connection between the state of the interior and atmospheric atmosphere. Therefore, understanding the whole life-cycle of a structure and composition. Current (TESS) and future (JWST, terrestrial planet – from its magma ocean origins to potentially a CHEOPS, PLATO) observatories will enhance our catalogue mature state with a solid mantle – has fundamental implications of rocky worlds through new discoveries and detailed charac- for the thermal and chemical evolution of terrestrial planets (e.g. terisation, particularly of atmospheres. These facilities demand Schaefer & Elkins-Tanton 2018). a concurrent advancement in modelling capabilities to extract Although definitions in the literature can vary, the magma maximum insight from observational data, particularly in terms ocean stage is typically defined as the period during which of the interplay between the interior, surface, and atmosphere. the planetary mantle has a melt fraction above a critical value, Article published by EDP Sciences A103, page 1 of 18 A&A 631, A103 (2019) usually between 30 and 50%. This critical value reflects the melt and composition of a planet due to inherent degeneracy that is fraction at which a partially molten mantle abruptly switches most appropriately addressed using a Bayesian framework (e.g. from a dynamic regime dominated by turbulent melt trans- Dorn et al. 2018). Additional data or modelling constraints can port to the comparatively slow viscous creep of solid materials. be used to limit the range of acceptable interior structures, such The boundary between these two regimes is known as the as a combination of stellar abundances (Dorn et al. 2016) and “rheological transition” or the “rheological front” since it is disk evolution models (Dorn et al. 2019). Ordinarily, static struc- strongly controlled by the phase and hence temperature depen- ture models assume an interior thermal structure, although this dency of viscosity (Solomatov 2000). For a magma ocean that is adopted a priori rather than relating directly to evolutionary crystallises from the bottom-up, this boundary moves upwards considerations. Planetary radii determined from static structure through the mantle from the core-mantle boundary to the surface models that consider only solid phases are relatively insensi- as the planet cools. tive to temperature (e.g. Dorn et al. 2018). However, temperature As inferred from solar system samples, the building blocks variations drive dynamic processes such as melting that facilitate of a rocky planet can contain several hundred ppm by mass of outgassing, which again provides a direct connection between volatiles that subsequently outgas as a magma ocean cools and the interior and potentially observable atmospheric signatures. crystallises. CO2 and H2O are the dominant gases released from An holistic modelling strategy is required to maximise our carbonaceous chondrites (Schaefer & Fegley 2010) and potent understanding of interior–atmosphere processes as future obser- greenhouse gases, and therefore they receive significant attention vational facilities push the limits of exoplanetary characterisa- in the context of coupled interior–atmosphere evolution (Elkins- tion. In this study, we highlight the potential to combine models Tanton 2008; Lebrun et al. 2013; Nikolaou et al. 2019). As a of coupled interior–atmosphere evolution with static structure magma ocean crystallises, the solubility of volatile species in calculations and modelled atmospheric spectra (transmission the melt phase drives outgassing as the solid fraction increases and emission). By combining these components in a common and the melt becomes over-saturated with volatiles. The growing modelling framework, we acknowledge planets as dynamic enti- atmosphere thermally insulates the magma ocean and thereby ties and leverage their evolution to bridge planet formation, dictates the early cooling rate, possibly extending the lifetime interior-atmosphere interaction, and observations. By consider- of a magma ocean from a few thousand years with no atmo- ing the earliest stage of terrestrial planet evolution – the magma sphere (e.g. Abe 1993) to several tens of million years with an ocean epoch – we investigate the consequences of large varia- atmosphere (e.g. Hamano et al. 2013). tions in temperature, material phase, and atmospheric properties For the terrestrial planets in the solar system, the magma on observations. This has immediate application for characteris- ocean stage was only transient, lasting at most a few hundred ing hot and close-in rocky planets. Ultimately, we wish to close million years. However, some exoplanets may harbour perma- the gap between atmospheric modelling and interior dynamics, nent magma oceans, which necessitates an understanding of the and thus pave the way for a new comprehension of the nature of spectral signature of magma oceans to assist in their character- rocky planets. isation. This is because there is an observational bias to detect exoplanets with short orbital periods, such that
Details
-
File Typepdf
-
Upload Time-
-
Content LanguagesEnglish
-
Upload UserAnonymous/Not logged-in
-
File Pages18 Page
-
File Size-