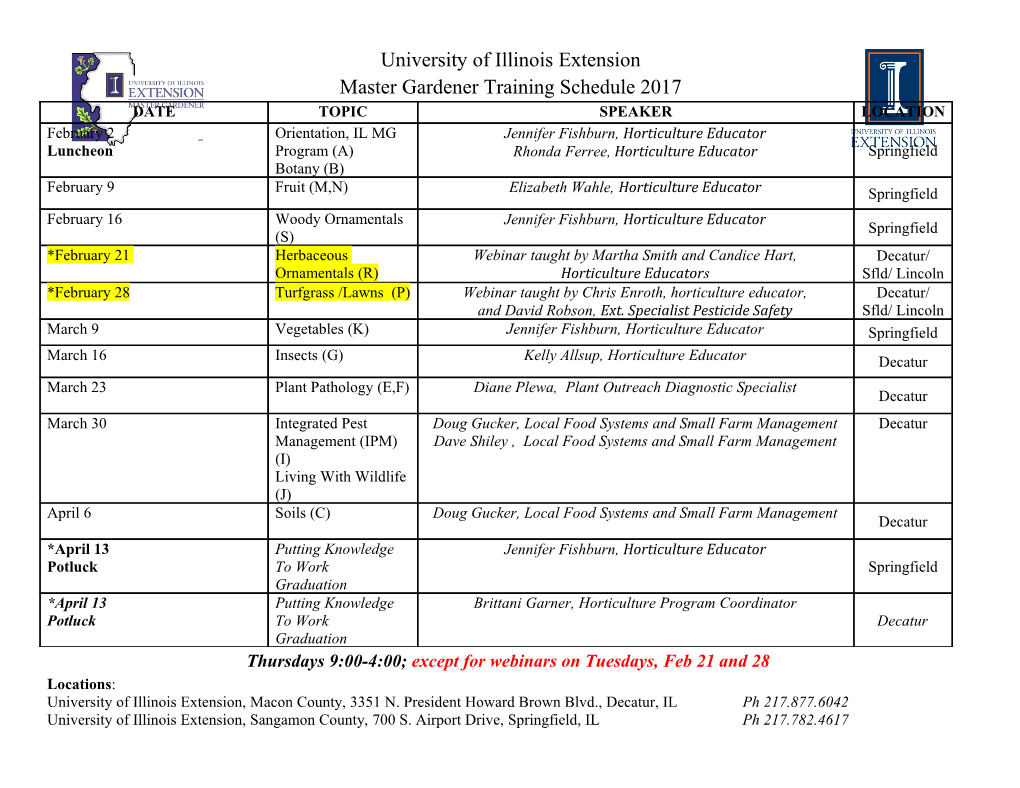
Upper Cretaceous sequences and sea-level history, New Jersey Coastal Plain Kenneth G. Miller² Department of Geological Sciences, Rutgers University, Piscataway, New Jersey 08854, USA Peter J. Sugarman New Jersey Geological Survey, P.O. Box 427, Trenton, New Jersey 08625, USA James V. Browning Department of Geological Sciences, Rutgers University, Piscataway, New Jersey 08854, USA Michelle A. Kominz Department of Geosciences, Western Michigan University, Kalamazoo, Michigan 49008-5150, USA Richard K. Olsson Mark D. Feigenson John C. HernaÂndez Department of Geological Sciences, Rutgers University, Piscataway, New Jersey 08854, USA ABSTRACT pean sections, and Russian platform BACKGROUND outcrops points to a global cause. Because We developed a Late Cretaceous sea- backstripping, seismicity, seismic strati- Predictable, recurring sequences bracketed level estimate from Upper Cretaceous se- graphic data, and sediment-distribution by unconformities comprise the building quences at Bass River and Ancora, New patterns all indicate minimal tectonic ef- blocks of the stratigraphic record. Exxon Pro- Jersey (ODP [Ocean Drilling Program] Leg fects on the New Jersey Coastal Plain, we duction Research Company (EPR) de®ned a 174AX). We dated 11±14 sequences by in- interpret that we have isolated a eustatic depositional sequence as a ``stratigraphic unit tegrating Sr isotope and biostratigraphy signature. The only known mechanism composed of a relatively conformable succes- (age resolution 60.5 m.y.) and then esti- that can explain such global changesÐ sion of genetically related strata and bounded mated paleoenvironmental changes within glacio-eustasyÐis consistent with forami- at its top and base by unconformities or their the sequences from lithofacies and biofacies niferal d18O data. Either continental ice correlative conformities'' (Mitchum et al., analyses. Sequences generally shallow up- sheets paced sea-level changes during the 1977, p. 53); by implication, ``genetically re- section from middle-neritic to inner-neritic Late Cretaceous, or our understanding of lated'' refers to global changes in sea level paleodepths, as shown by the transition causal mechanisms for global sea-level (Vail et al., 1977). Christie-Blick and Driscoll from thin basal glauconite shelf sands change is fundamentally ¯awed. Compari- (1995) clari®ed the genetic connotation by (transgressive systems tracts [TST]), to son of our eustatic history with published recognizing sequence boundaries as unconfor- medial-prodelta silty clays (highstand sys- ice-sheet models and Milankovitch predic- mities associated with lowering of base level, tems tracts [HST]), and ®nally to upper± tions suggests that small (5±10 3 106 km3), including eustatic and tectonic mechanisms. delta-front quartz sands (HST). Sea-level ephemeral, and areally restricted Antarctic Sequences have been recognized in diverse estimates obtained by backstripping (ac- ice sheets paced the Late Cretaceous global stratigraphic environments (e.g., ranging from counting for paleodepth variations, sedi- sea-level change. New Jersey and Russian siliciclastic to carbonate settings; see exam- ment loading, compaction, and basin sub- eustatic estimates are typically one-half of ples in Wilgus et al., 1988; de Graciansky et sidence) indicate that large (.25 m) and the EPR amplitudes, though this difference al., 1998) from the Proterozoic (e.g., Christie- rapid (K1 m.y.) sea-level variations oc- varies through time, yielding markedly dif- Blick et al., 1988) to the Holocene (e.g., Lock- curred during the Late Cretaceous green- ferent eustatic curves. We conclude that er et al., 1996) and have been related to global house world. The fact that the timing of Up- New Jersey provides the best available es- sea-level (eustatic) variations (Vail et al., per Cretaceous sequence boundaries in timate for Late Cretaceous sea-level 1977; Haq et al., 1987; Posamentier et al., New Jersey is similar to the sea-level low- variations. 1988). However, differences in accommoda- ering records of Exxon Production Re- tion (the sum of subsidence and eustatic search Company (EPR), northwest Euro- Keywords: eustasy, sequence stratigraphy, change) and sediment supply control sequence sea-level history, New Jersey Coastal Plain, architecture, including the nature and signi®- ²E-mail: [email protected]. Late Cretaceous, backstripping. cance of surfaces bounding and within se- GSA Bulletin; March/April 2004; v. 116; no. 3/4; p. 368±393; doi: 10.1130/B25279.1; 13 ®gures; 1 table; Data Repository item 2004043. For permission to copy, contact [email protected] 368 q 2004 Geological Society of America UPPER CRETACEOUS SEQUENCES AND SEA-LEVEL HISTORY, NEW JERSEY COASTAL PLAIN dle Eocene (Barron, 1983; Huber et al., 2002), the only known mechanism for producing the large (k10 m), rapid (,1 m.y.) global sea- level changes reported for this time (e.g., Haq et al., 1987; Hallam, 1992) is glacio-eustasy (see Donovan and Jones, 1979; Pitman and Golovchenko, 1983). Studies have begun to question the assumption of a completely ice- free world during the Cretaceous (e.g., Stoll and Schrag, 1996; Miller et al., 1999a, 2002; Price, 1999), suggesting that ice-volume changes may have been an important control on greenhouse sea-level changes. Evaluation of mechanisms of sea-level change requires a ®rm chronology and a means of extracting rates of change. Several passive-margin and epicontinental sea regions provide relatively high-resolution sequence- stratigraphic records for the Late Cretaceous. Deposits of the U.S. Western Interior Seaway provide a tephrochronology of sequences (Kauffman, 1977; Dean and Arthur, 1998), though these sections are complicated by com- pressional tectonics. The epicontinental and passive-margin sections of northwest Europe and the Russian platform provide excellent records of transgressive-regressive sequences (Hancock, 1993; de Graciansky et al., 1998; Sahagian et al., 1996). However, differing bio- zonal schemes complicate interregional cor- relations, and a ®rm global record of Upper Cretaceous sequences has proved to be elu- sive. Though workers at EPR produced a Late Cretaceous eustatic record (Haq et al., 1987), the database on which it is published is largely proprietary. De Graciansky et al. (1998) have provided public documentation of EPR's Me- sozoic sequences in northwest Europe. Where- as their Lower Cretaceous and older sequenc- es are reasonably well documented, the Upper Cretaceous sequences are poorly constrained in age. Pieces of the Upper Cretaceous Figure 1. Map showing the New Jersey Coastal Plain, the limit of Miocene and younger sequence-stratigraphic puzzle are falling into outcrop that approximates strike, and sites discussed here. place with publication of detailed studies of parts of the section (e.g., Gale et al. [2002] for the Cenomanian±early Turonian), but the quences (e.g., ¯ooding surfaces, transgressive shows that sequence boundaries from 42 to 8 overall picture of Upper Cretaceous sequences surfaces). In addition, accommodation and Ma correlate with global d18O increases, link- and rates of sea-level change is still blurry. sediment supply control the stacking patterns ing them with glacio-eustatic sea-level low- The New Jersey passive margin, particular- of facies successions within sequences (e.g., erings (Miller et al., 1996, 1998a). However, ly the onshore coastal plain (Fig. 1), has pro- systems tracts of Vail et al., 1977, and Posa- such a relationship has not been established vided a reference for Cenozoic sequences mentier et al., 1988) and the general three- for the ``greenhouse world'' of the pre±middle (Miller et al., 1996, 1998a) and potentially can dimensional arrangement of sequences (Rey- Eocene, in part re¯ecting the greater dif®culty provide similar records of Upper Cretaceous nolds et al., 1991). in obtaining and dating sequences older than sequences. The development of Late Creta- A clear relationship between sequence 42 Ma from passive margins throughout the ceous Sr isotope stratigraphy (Fig. 2; Burke et boundaries and glacio-eustatic sea-level low- world. al., 1982; Sugarman et al., 1995; McArthur et erings has been demonstrated for the ``ice- Mechanisms for sea-level change during the al., 1992, 1993, 1994; Howarth and McArthur, house world'' of the past 42 m.y. (Miller et greenhouse world are poorly understood. 1997) together with the application of Creta- al., 1996, 1998a). Comparing onshore and off- Though most investigators have assumed that ceous nannoplankton biostratigraphy (e.g., shore New Jersey sequences with d18O records Earth was largely ice free prior to the late mid- Bralower et al., 1995) provides the means of Geological Society of America Bulletin, March/April 2004 369 MILLER et al. Figure 2. Later Cretaceous Sr isotope age calibration for ODP (Ocean Drilling Program) Site 525 (open circles), U.S. Western Interior (open squares), and Kronsmoor, Germany (closed circles). A ®fth-order polynomial (thin gray line) and two linear ®ts (thick black lines) are shown. reconciling correlations among regions and m paleodepth), prodelta silts (20±60 m), and ance on cuttings or discontinuous cores, or the evaluating the global signi®cance of regional outer-neritic glauconite sands (60±200 m). far updip locations of boreholes. sequences. Previous studies have documented at least Continuous coring and logging by onshore ®ve to eight Late Cretaceous cycles. Olsson ODP Leg 174AX at Bass River and Ancora, UPPER CRETACEOUS SEQUENCES IN (1963, 1975) recognized
Details
-
File Typepdf
-
Upload Time-
-
Content LanguagesEnglish
-
Upload UserAnonymous/Not logged-in
-
File Pages26 Page
-
File Size-