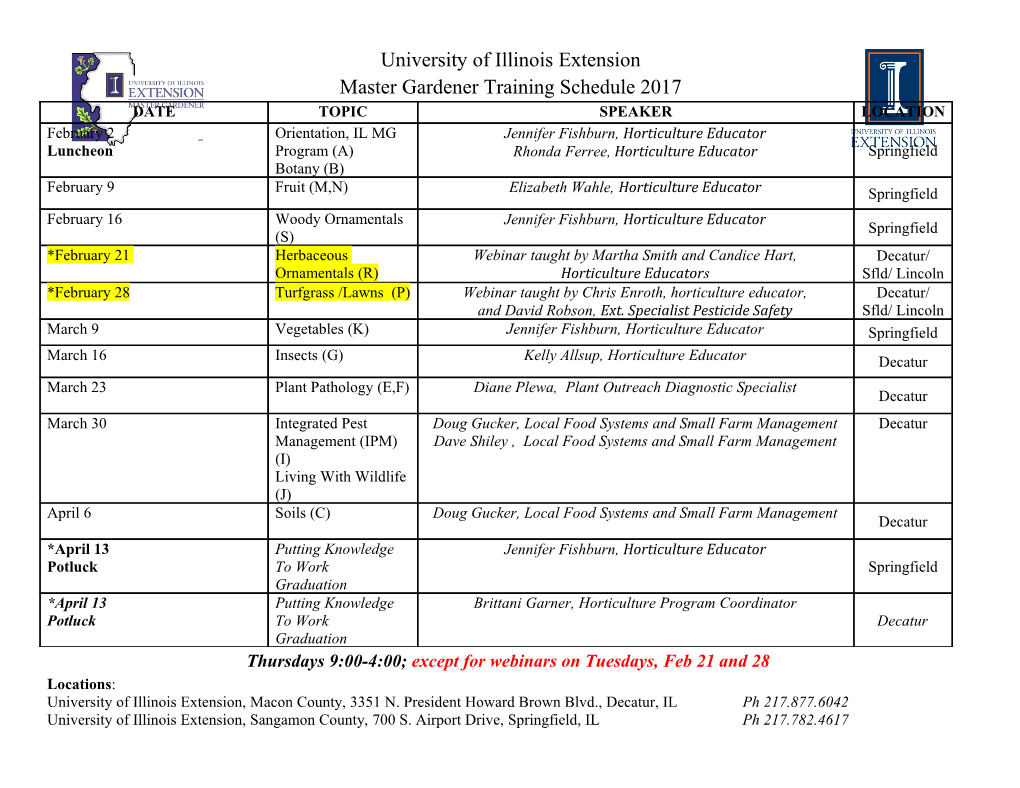
New Research Cognition and Behavior Trace Eyeblink Conditioning in Mice Is Dependent upon the Dorsal Medial Prefrontal Cortex, Cerebellum, and Amygdala: Behavioral Characterization and Functional Circuitry1,2,3 Richard Gray,1 Brian Kalmbach,1 Boris V. Zemelman,1,2 Niraj ء,William Taylor,1 ء,Jennifer J. Siegel,1 S. Desai,1 Daniel Johnston,1,2 and Raymond A. Chitwood1,2 DOI:http://dx.doi.org/10.1523/ENEURO.0051-14.2015 1Center for Learning and Memory, University of Texas at Austin, Austin, Texas 78712, and 2Department of Neuroscience, University of Texas at Austin, Austin, Texas 78712 Abstract Trace eyeblink conditioning is useful for studying the interaction of multiple brain areas in learning and memory. The goal of the current work was to determine whether trace eyeblink conditioning could be established in a mouse model in the absence of elicited startle responses and the brain circuitry that supports this learning. We show here that mice can acquire trace conditioned responses (tCRs) devoid of startle while head-restrained and permitted to freely run on a wheel. Most mice (75%) could learn with a trace interval of 250 ms. Because tCRs were not contaminated with startle-associated components, we were able to document the development and timing of tCRs in mice, as well as their long-term retention (at 7 and 14 d) and flexible expression (extinction and reacquisition). To identify the circuitry involved, we made restricted lesions of the medial prefrontal cortex (mPFC) and found that learning was prevented. Furthermore, inactivation of the cerebellum with muscimol completely abolished tCRs, demonstrating that learned responses were driven by the cerebellum. Finally, inactivation of the mPFC and amygdala in trained animals nearly abolished tCRs. Anatomical data from these critical regions showed that mPFC and amygdala both project to the rostral basilar pons and overlap with eyelid-associated pontocerebellar neurons. The data provide the first report of trace eyeblink conditioning in mice in which tCRs were driven by the cerebellum and required a localized region of mPFC for acquisition. The data further reveal a specific role for the amygdala as providing a conditioned stimulus-associated input to the cerebellum. Key words: anterograde labeling; cerebellum; pontine nuclei; prefrontal cortex; retrograde labeling; trace eye- blink conditioning Significance Statement The current study characterizes the learned behavior and identifies the brain circuitry necessary for trace eyeblink conditioning in mice. We report that under learning conditions designed to avoid elicited startle responses, mice expressed learned responses that were cerebellar-dependent and required a localized region of the dorsal medial prefrontal cortex. Using functional inactivation paired with anatomical tracers, we further identify the trace eyeblink conditioning circuitry in the mouse model that underlies this basic associative learning task, including a more refined role for how amygdala inputs might support this task in the absence of conditioned stimulus-evoked startle. July/August 2015, 2(4) e0051-14.2015 1–29 New Research 2 of 29 Introduction stimuli in the basilar pons, a source of forebrain input to Awake behaving mice offer many advantages for study- the cerebellum, and has been suggested to drive faster ing higher cognitive function. However, it can be challeng- acquisition of the CR (Taub and Mintz, 2010). However, ing to develop associative learning and memory tasks in many eyeblink studies in rodents that used a tone CS rodents without also engaging startle circuitry, particularly noted evidence of acoustic startle in eyelid responses with the use of acoustic stimuli. Rodents show pro- during at least some trials (Takatsuki et al., 2001; Tseng nounced acoustic startle, which results in a fast motor et al., 2004; Ewers et al., 2006; Brown et al., 2010), and so response (Ͻ50 ms) that includes the facial muscles (Koch, the putative role of the amygdala may more accurately 1999). Additionally, startle responses can be either poten- reflect an interaction between startle and eyeblink cir- tiated or habituated with experience, complicating analy- cuitry. To further complicate matters, it has also been sis of learned behaviors that result from training. The shown that the amygdala can drive a short latency non- potentiation of startle is mediated by amygdala activation cerebellar learned eyelid response (onset at 50–70 ms, (Rosen and Davis, 1988), and field potential recordings peaking at ϳ115 ms) that occurs in parallel and overlaps from the amygdala show excitatory responses in associ- with the well timed cerebellar component (Boele et al., ation with the expression of startle (Ebert and Koch, 2010; Sakamoto and Endo, 2010). The two different re- 1997). sponses can be dissociated by inactivation of either the One example of an associative learning task that often cerebellum or amygdala, leaving the residual response uses an acoustic stimulus is eyeblink conditioning—a intact (Koekkoek et al., 2005; Sakamoto and Endo, 2010). form of associational learning that pairs a neutral condi- Given that both the mPFC and hippocampus have been tioning stimulus (CS) with a reflexive unconditioned stim- shown to interact with the amygdala, it becomes difficult ulus (US) to elicit an eyeblink. With repeated pairings, to determine how these brain regions might support trace animals learn to close the eyelid in response to the CS and eyeblink conditioning in rodents when overlapping brain in anticipation of the US (the conditioned response; CR). systems are being engaged. Two variants of eyeblink conditioning, trace and delay Recently, Medina and colleagues (Chettih et al., 2011; differ in the temporal relationship between the CS and US, Heiney et al., 2014) reported modified training procedures and in the underlying brain circuitry. Trace conditioning for delay eyeblink conditioning in mice in which acoustic includes a stimulus-free interval between the CS and US, startle is avoided by using a blue LED as a CS, and mice whereas the stimuli overlap in delay conditioning. Al- are permitted to freely run while head-fixed on a wheel though both trace and delay conditioning require the allowing for direct and detailed measurement of eyelid cerebellum to drive motor output, trace conditioning has movements with high-speed cameras. By implementing been shown to also require forebrain regions, such as the those procedures during trace eyeblink conditioning, we medial prefrontal cortex (mPFC) and hippocampus (Stein- show here that mice can acquire trace CRs in the absence metz et al., 1989; Kim et al., 1995; Kronforst-Collins and of startle and which are completely abolished with cere- Disterhoft, 1998; Weiss et al., 1999; Takehara et al., 2003; bellar inactivation. In contrast to previous observations, Tseng et al., 2004; Kalmbach et al., 2009, 2010b; Siegel amygdala inactivation also resulted in CR abolishment, and Mauk, 2013; Chen et al., 2014). suggesting that under these conditions the amygdala may In rodents, however, the amygdala may also play an provide an input to the basilar pons that is part of the CS essential role in eyeblink conditioning (Boele et al., 2010). representation. Using lesions, we identified a restricted For example, lesions or inactivation of the amygdala re- region within the mPFC necessary for the acquisition of sulted in slower acquisition of delay CRs and deficits in CRs, and substantiated that a 250 ms trace interval is asymptotic performance in both rats and mice (Lee and indeed forebrain-dependent in mice. The data presented Kim, 2004; Sakamoto and Endo, 2010). Amygdala activa- here provide the first report of trace CRs in mice whose tion has been shown to facilitate neural responses to acquisition is mPFC-dependent and for which the motor response is driven by the cerebellum. Received November 4, 2014; accepted June 26, 2015; First published July 02, 2015. Materials and Methods 1The authors report no conflicts of interest. 2Author Contributions: J.J.S., R.A.C., B.K., and D.J. designed research; Subjects and surgical procedures J.J.S. and W.T. performed research; R.G. and N.S.D. contributed unpublished All surgical and experimental procedures were ap- acquisition and analytic tools; B.V.Z. contributed viral tools; J.J.S., W.T., proved and performed according to the regulations of the R.A.C.. and N.S.D. analyzed the data; J.J.S., W.T., R.A.C., D.J., R.G., B.V.Z., authors University’s Institutional Animal Care and Use B.K., and N.S.D. wrote the paper and constructed figures 3This work was supported by the National Institutes of Health RO1 Committee and were in accordance with the National MH094839 to D.J. and Michael D. Mauk, and the McKnight Foundation. Institutes of Health guidelines. Male C57BL/6 mice (10– *J.J.S. and W.T. contributed equally to this work. 14-weeks-old, The Jackson Laboratory) were shifted to a Correspondence should be addressed to Dr. Jennifer J. Siegel, Center for reverse dark cycle (lights off at 9:00 A.M. and lights on at Learning & Memory, University of Texas at Austin, 1 University Station Stop 9:00 P.M.), at least 2 weeks prior to surgery and remained C7000, Austin, TX 78712-0805. E-mail: [email protected]. DOI:http://dx.doi.org/10.1523/ENEURO.0051-14.2015 on this cycle throughout behavioral training. All experi- Copyright © 2015 Siegel et al. ments were performed during the mice’s dark, normally This is an open-access article distributed under the terms of the Creative active, period. Both the animal housing facility and the Commons Attribution 4.0 International, which permits unrestricted use, distri- bution and reproduction in any medium provided that the original work is room where training took place were outfitted with red properly attributed. lights in order not to disrupt the reversed light/dark cycle. July/August 2015, 2(4) e0051-14.2015 eNeuro.sfn.org New Research 3 of 29 Training was restricted to between 9:00 A.M.
Details
-
File Typepdf
-
Upload Time-
-
Content LanguagesEnglish
-
Upload UserAnonymous/Not logged-in
-
File Pages29 Page
-
File Size-