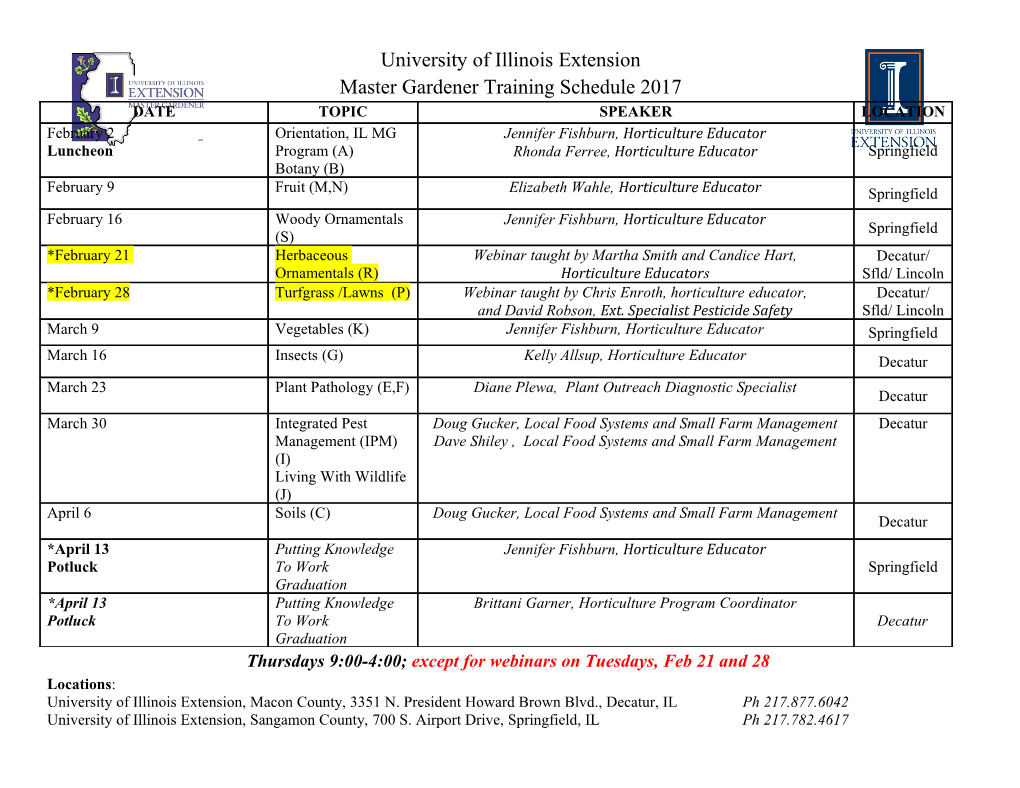
Structural and functional insights into apicomplexan gliding and its regulation Dissertation to obtain the degree of Doctor of Natural Sciences University of Hamburg Faculty of Mathematics, Informatics and Natural Sciences at the Department of Biology by Samuel Pažický from Bratislava, Slovakia Hamburg 2020 Examination commission Examination commission chair Prof. Dr. Jörg Ganzhorn (University of Hamburg) Examination commission members Prof. Jonas Schmidt-Chanasit (Bernhard Nocht Institute for Tropical Medicine and University of Hamburg) Prof. Tim Gilberger (Bernhard Nocht Institute for Tropical Medicine, Centre for Structural Systems Biology and University of Hamburg) Dr. Maria Garcia-Alai (European Molecular Biology Laboratory and Centre for Structural Systems Biology) Dr. Christian Löw (European Molecular Biology Laboratory and Centre for Structural Systems Biology) Date of defence: 29.01.2021 This work was performed at European Molecular Biology Laboratory, Hamburg Unit under the supervision of Dr. Christian Löw and Prof. Tim-Wolf Gilberger. The work was supported by the Joachim Herz Foundation. Evaluation Prof. Dr. rer. nat. Tim-Wolf Gilberger Bernhard Nocht Institute for Tropical Medicine (BNITM) Department of Cellular Parasitology Hamburg Dr. Christian Löw European Molecular Biology Laboratory Hamburg unit Hamburg Prof. Dr. vet. med. Thomas Krey Hannover Medical School Institute of Virology Declaration of academic honesty I hereby declare, on oath, that I have written the present dissertation by my own and have not used other than the acknowledged resources and aids. Eidesstattliche Erklärung Hiermit erkläre ich an Eides statt, dass ich die vorliegende Dissertationsschrift selbst verfasst und keine anderen als die angegebenen Quellen und Hilfsmittel benutzt habe. Hamburg, 22.9.2020 Samuel Pažický List of contents Declaration of academic honesty 4 List of contents 5 Acknowledgements 6 Summary 7 Zusammenfassung 10 List of publications 12 Scientific contribution to the manuscript 14 Abbreviations 16 1. Introduction 18 1.1. Apicomplexa 18 1.1.1. Classification 18 1.1.2. Plasmodium falciparum life cycle 19 1.1.3. Toxoplasma gondii life cycle 21 1.2. Morphology of motile apicomplexan stages 22 1.2.1. Secretory organelles of zoites 23 1.3. Gliding motility and host cell invasion 25 1.3.1. Surface molecules in gliding and invasion 25 1.3.2. The invasion motor 27 2. Discussion 33 2.1. Structure of the glideosome 33 2.1.1. Structure of MyoA with bound light chains 33 2.1.2. Impact of phosphorylation on ELC binding 35 2.1.3. Role of ELCs in the regulation of the glideosome 36 2.1.4. Role of calcium in the glideosome regulation 37 2.1.5. Glideosome associated proteins 38 2.1.6. Unidentified glideosome members 39 2.1.7. Towards the full glideosome structure 39 2.2. Drug against malaria and the glideosome as a drug target 40 2.2.1. Antimalarial compounds 40 2.2.2. Drugs directed against the glideosome 42 2.2.3. Drug targets upstream of the glideosome and regulation of the gliding motility 45 2.3. Regulation of PfGSK3 49 2.4. Rhoptries and their biogenesis 51 3. Outlook and concluding remarks 54 4. Bibliography 56 5. Manuscript 1 71 6. Manuscript 2 127 7. Manuscript 3 155 Acknowledgements First, I would like to thank Dr. Christian Löw for giving me the opportunity to work in his laboratory. As a mentor, he always found a time to consult my results, provide me with a valuable feedback and steer the work in the right direction. With his scientific excellence and great organizational skills, he helped me to improve as a scientist and as a person, too. My thanks also go to my thesis advisory committee members: Dr. Orsolya Barabas, Dr. Jan Kosinski and Prof Tim Gilberger, whose advice always turned my project in the right direction. Special thanks go to the scientists that directly contributed to my project: Dr. Ulrich Weininger, Dr. Arne Alder, Dr. Karol Kaszuba and Dr. Haydyn Mertens. I would also like to thank (Dr.-to-be) Karthikeyan Dhamotharan who I had a chance to mentor for one year. His dedication, precision and fast learning crucially helped with the progress of the project and I am very thankful for all his hard work. I would also like to acknowledge all the current and past members of the Löw group, who have not only given me infinite amount of scientific advice, but also have become my good friends. At the same time, I am very thankful for the community at EMBL Hamburg, the wet lab team, SPC team, IT group, beamline scientists and all fellow predocs, postdocs and scientists, without whom I would not be able push the project so far. Thank you for making EMBL a special place. Not least, I want to thank Samantha Seah for her tremendous support and for the amazing journey that we went through over the last four years. My thanks also go to my closest friends who have always been there for me in spite of the long distance. Finally, I would like to thank my family. Without their unprecedented love and support, I would not have become the person that I am now. 6 Summary Apicomplexans are a group of single cell parasitic organisms that are infectious agents of many human and animal diseases. For example, Plasmodium species cause malaria, which infects over 200 million and kills almost 400 thousands people every year. Toxoplasma species have infected about 30-50% of the entire population. While infection is benign for most, it is a threat for immunocompromised patients and pregnant women. Several other apicomplexan species are causative agents of diseases in domesticated animals in Africa, representing further financial and humanitarian burdens. A typical apicomplexan parasite completes a sophisticated life cycle, usually switching between two host organisms to engage in sexual reproduction in one and the asexual reproduction in the other. During their life cycle, the parasites differentiate into distinct life stages that possess unique shapes and morphologies. Motile life stages, in which the parasites move through the tissues and invade their host cells, are of specific interest because the parasite cells are exposed to the extracellular environment outside the host cells. Consequently, they are more accessible to the host immune system and drugs, making them and their proteins major drug targets. To move across tissues and invade host cells, apicomplexans use a specific type of motility that does not require a change in their cell shape, called gliding. Many organelles and subcellular structures of the motile apicomplexan stages are designed to mediate efficient gliding and host cell invasion. These parasitic cells are elongated and polarized, forming an apical pole in the anterior and basal end in the posterior of the parasite. The parasites glide and invade the host cell in the apical direction. The apical pole contains specialized secretory organelles, such as micronemes and rhoptries, that discharge important virulence factors essential for gliding and for host cell invasion. Other specialized organelles, alveoli, also called inner membrane complex (IMC), form a network of large flat vesicles underneath the parasite`s plasma membrane to accommodate the molecular motor that powers the motility and invasion process. This thesis cumulates three separate bodies of work that represent advances in understanding of the processes that are important in gliding motility and host cell invasion by apicomplexan parasites. The first work (manuscript 1) reveals the structure and the function of the essential light chains (ELCs) of Plasmodium falciparum and Toxoplasma gondii. In apicomplexan parasites, ELCs are part of the glideosome, a larger complex residing between the parasite`s plasma membrane and the IMC. The glideosome consists of a myosin motor protein MyoA, two light chains – ELC and myosin light chain 1 (MLC1) and three glideosome-associated proteins (GAPs). It has been shown that ELCs bind the neck 7 domain of MyoA and facilitate an enhanced motor efficiency. However, the molecular mechanism behind this phenomenon remains unclear. We have shown that the two T. gondii ELCs (TgELC1 and TgELC2) as well as P. falciparum ELC (PfELC) bind a conserved sequence of the MyoA neck domain. We have determined the structures of all ELCs bound in trimeric complexes with their respective MyoA neck domains and MLC1s, representing the first glideosome trimeric complexes shown so far. These structures reveal that the C-terminus of PfELC, which is partially unfolded in an unbound state, maintains some degree of flexibility even in the complex, resulting in a different interaction angle compared to TgELCs and explaining lower affinity of PfELC to MyoA compared to its T. gondii analogues. Additionally, we have shed a light on the regulatory function of ELCs, showing that two phosphorylation sites can regulate their binding to MyoA. On the other hand, we proved that the binding of calcium does not regulate the ELC binding and only increases the stability of the trimeric complexes. Finally, we have shown that upon binding, the ELCs induce a formation of secondary structure in MyoA neck, leading to a stiffer MyoA neck that consequently serves more efficiently as a lever arm and increases the motor speed. In the second project (manuscript 2), we have tackled the role of a rhoptry protein Armadillo Repeat- Only (ARO) and its putative interaction partner, ARO interacting protein (AIP). ARO is anchored to the membrane of the rhoptries from their cytoplasmic side by palmitoylation and myristoylation. In T. gondii, ARO has been shown to be essential for the correct positioning of rhoptries in the apical pole and to interact with AIP1, myosin F (MyoF) and adenylate cyclase beta (ACβ). However, it was not clear whether the same arrangement holds true in P. falciparum. We solved the crystal structure of PfARO that, with five armadillo repeat motifs, assumes a bean-shaped conformation. We identified the P.
Details
-
File Typepdf
-
Upload Time-
-
Content LanguagesEnglish
-
Upload UserAnonymous/Not logged-in
-
File Pages185 Page
-
File Size-