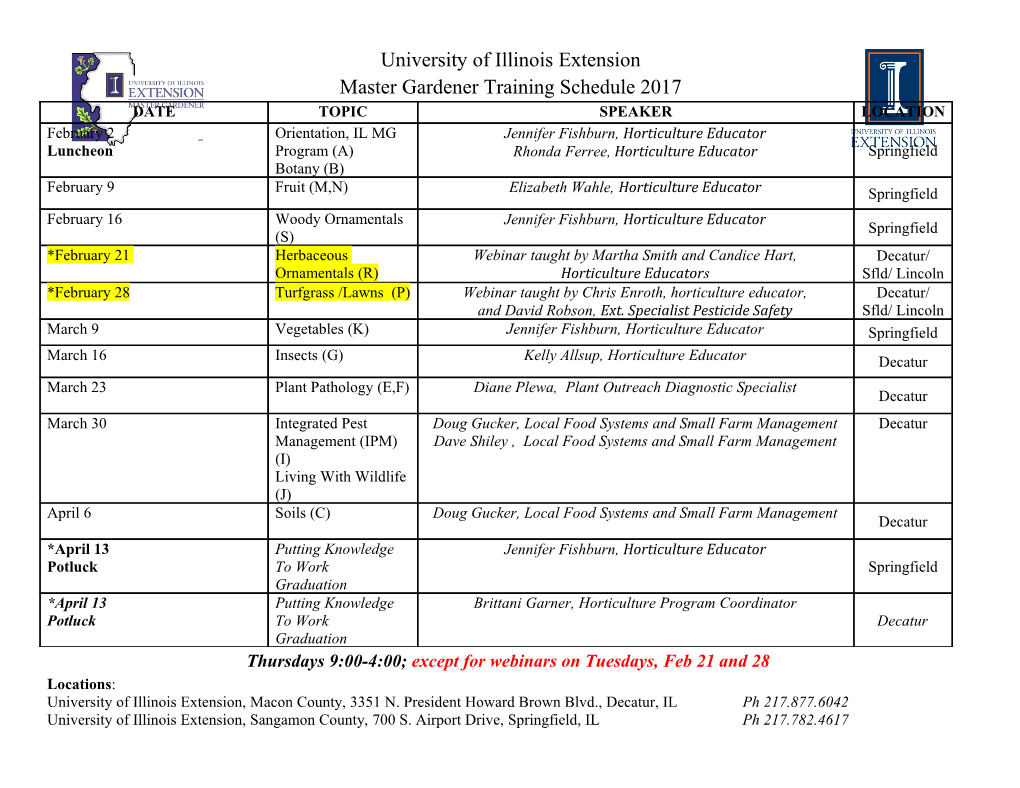
REVIEW GENETIC TOOLBOX Tetrahymena as a Unicellular Model Eukaryote: Genetic and Genomic Tools Marisa D. Ruehle,*,1 Eduardo Orias,† and Chad G. Pearson* *Department of Cell and Developmental Biology, University of Colorado Anschutz Medical Campus, Aurora, Colorado, 80045, and †Department of Molecular, Cellular, and Developmental Biology, University of California, Santa Barbara, California 93106 ABSTRACT Tetrahymena thermophila is a ciliate model organism whose study has led to important discoveries and insights into both conserved and divergent biological processes. In this review, we describe the tools for the use of Tetrahymena as a model eukaryote, including an overview of its life cycle, orientation to its evolutionary roots, and methodological approaches to forward and reverse genetics. Recent genomic tools have expanded Tetrahymena’s utility as a genetic model system. With the unique advantages that Tetrahymena provide, we argue that it will continue to be a model organism of choice. KEYWORDS Tetrahymena thermophila; ciliates; model organism; genetics; amitosis; somatic polyploidy ENETIC model systems have a long-standing history as cost-effective laboratory handling, and its accessibility to both Gimportant tools to discover novel genes and processes in forward and reverse genetics. Despite its affectionate reference cell and developmental biology. The ciliate Tetrahymena ther- as “pond scum” (Blackburn 2010), the beauty of Tetrahymena mophila is a model system that combines the power of for- as a genetic model organism is displayed in many lights. ward and reverse genetics with a suite of useful biochemical Tetrahymena has a long and distinguished history in the and cell biological attributes. Moreover, Tetrahymena are discovery of broad biological paradigms (Figure 2), begin- evolutionarily divergent from the commonly studied organ- ning with the discovery of the first microtubule motor, dynein isms in the opisthokont lineage, permitting examination of (Gibbons and Rowe 1965). Others include the Nobel Prize both unique and universally conserved biological processes. winning discoveries of catalytic RNA (Kruger et al. 1982) and Here we highlight the advantages of Tetrahymena as a model telomere structure and telomerase (Greider and Blackburn system, such as its unique and easily manipulated life cycle, 1985). The first histone-modifying enzyme (histone acetyl that have contributed to important discoveries. The growing transferase) and its role as a transcription factor were discov- suite of molecular-genetic and genomic tools described here ered in Tetrahymena (Brownell et al. 1996), which gave birth provides a system to couple gene discovery to mechanistic to the “histone code” and the field of epigenetic control of dissections of gene function in the cell. gene expression by chromatin modification. The role of small T. thermophila (Figure 1) is a ciliate model organism interfering RNAs in heterochromatin formation and the mas- whose study has led to fundamental biological insights cov- sive, programmed excision of transposon-related DNA from ering the central dogma and beyond. Indeed, this single- the somatic genome (Mochizuki et al. 2002; Taverna et al. celled, motile eukaryote provides the tools and techniques 2002) is another major Tetrahymena contribution. These fun- not only for novel gene discovery, but also for unveiling the damental discoveries in Tetrahymena have helped usher in ’ important molecular mechanisms behind those genes func- the modern era of molecular and cellular biology. Many of the ’ tions. As such, Tetrahymena s utility as a genetic model reasons why Tetrahymena was an advantageous system for organism is revealed by its short life cycle, easy and these groundbreaking discoveries are the same that make Tetrahymena useful today and in the future, augmented by the ever-expanding toolkit available to Tetrahymena Copyright © 2016 by the Genetics Society of America doi: 10.1534/genetics.114.169748 researchers. Manuscript received March 1, 2016; accepted for publication April 8, 2016. In this review, we discuss major biological questions to 1Corresponding author: Department of Cell and Developmental Biology, University of Colorado Denver School of Medicine, 12801 E. 17th Ave., Mail Stop 8108, Aurora, which T. thermophila is amenable and the genetic tools avail- CO 80045. E-mail: [email protected] able to answer them. It begins with the general biology of Genetics, Vol. 203, 649–665 June 2016 649 genome consists of 200 different chromosomes, each main- tained at a ploidy of 45 during the G1 phase of the cell cycle. The MIC is transcriptionally silent, and all gene expression is driven from the MAC DNA. Despite the fact that Tetrahymena are unicellular organisms, this germline/soma separation is reminiscent of metazoans where distinct germ cells and so- matic cells are maintained. Germline/soma separation is ex- tremely rare among unicellular eukaryotes, though it is also found in certain Foraminifera (in the Rhizarian evolutionary group). To replicate these distinct genomes and to maintain ge- netic diversity, the T. thermophila life cycle employs both asex- ual, vegetative cell division and sexual reorganization through a process called conjugation. During vegetative division, the MAC divides amitotically (random chromosome segregation), the MIC divides mitotically, and binary cell fission produces two daughter cells. However, under conditions of starvation, pairs of sexually mature cells with different mating types un- dergo conjugation. T. thermophila cells can have one of seven different mating types, and each mating type can conjugate with another mating type, but not with itself (Elliott and Gruchy 1952; Nanney and Caughey 1953). Conjugation in- volves highly conserved eukaryotic processes: meiosis, game- Figure 1 Tetrahymena cell image, illustrating its crystal-like organization togenesis, and gamete nucleus fusion to form the fertilization of ciliary units. A single Tetrahymena cell labeled for basal bodies (a-centrin, (or “zygote”) nuclei of progeny cells (Figure 3). The fertiliza- et al. fi red) (Stemm-Wolf 2005), kinetodesmal bers (5D8, green) (Jerka- tion nucleus divides mitotically and then differentiates into a Dziadosz et al. 1995), and DNA (Hoerscht-33342, blue). Note that basal bodies are packed together in the four ciliary “membranelles” of the oral new MIC as well as a new MAC, and the parental MAC is apparatus, for which the genus is named. Bar, 10 mm. eliminated. Conjugation has important consequences: Mende- lian genetic inheritance and increasing genotypic diversity.The genome’s maintenance and expression depend on widely con- Tetrahymena and its unique advantages as an experimental served mechanisms and pathways across all lineages of life, model system. We then describe both forward and reverse making Tetrahymena a useful organism in which to study these genetic strategies in Tetrahymena to facilitate gene discovery universal processes. and to interrogate the mechanistic underpinnings of those Evolutionary context genes. Ultimately, we seek to engage future researchers by describing the wealth of experimental advantages (both his- As ciliates, Tetrahymena are a part of the Alveolate group of torical and modern) that Tetrahymena can provide and pre- the Stramenopile–Alveolate–Rhizarian (SAR) lineage (Fig- view its promising future. ure 4). Dinoflagellates and apicomplexans are also a part of the Alveolate group, but are more closely related to each other than to the ciliates. Thus, there is a large evolutionary Tetrahymena Biology distance from ciliates to their sister Alveolate groups, and an Tetrahymena are unicellular, ciliated eukaryotes that live in even greater distance to other commonly used eukaryotic model fresh water over a wide range of conditions. In the wild, organisms which, like humans, are a part of the opisthokont Tetrahymena feed on bacteria, but laboratory strains typically group (yeast, worms, flies, mice, and many others). There is a live as axenic cultures in nutrient-rich media (derived from noticeable dearth of model organisms outside of the opisthokont tissue extracts) or chemically defined media. Populations group, making research of nonopisthokonts such as Tetrahy- grow quickly, with cells dividing every 2–3 hr under optimal mena an attractive means to explore the remarkable diversity conditions. Each cell is large, 30–50 mminlength,mak- of eukaryotic cell biology (Lynch et al. 2014). Nevertheless, ing them ideal for light and electron microscopy-based Tetrahymena utilize many universally conserved eukaryotic investigations. processes, making them also useful for illuminating these Tetrahymena, like all ciliates, are nuclear dimorphic, conserved features (Briguglio and Turkewitz 2014; Lynch which means that the germline genome and the somatic ge- et al. 2014). nome exist within two separate nuclei in one cell: the micro- Tetrahymena’s closest model organism relative (though nucleus (MIC) and the macronucleus (MAC), respectively not its closest relative outright) is Paramecium, which, along (Table 1, Table 2, and Figure 1). The diploid MIC genome with Tetrahymena, is a part of the Oligohymenophorea class consists of five pairs of chromosomes, whereas the MAC (Figure 4, bottom). However, based on deviations in small 650 M. D. Ruehle, E. Orias, and C. G. Pearson and later T. pyriformis syngen 1, before receiving its current name (Nanney and McCoy 1976). Isogenic, inbred lines of T. thermophila have been derived (Allen 1967). By conven- tion, most
Details
-
File Typepdf
-
Upload Time-
-
Content LanguagesEnglish
-
Upload UserAnonymous/Not logged-in
-
File Pages17 Page
-
File Size-