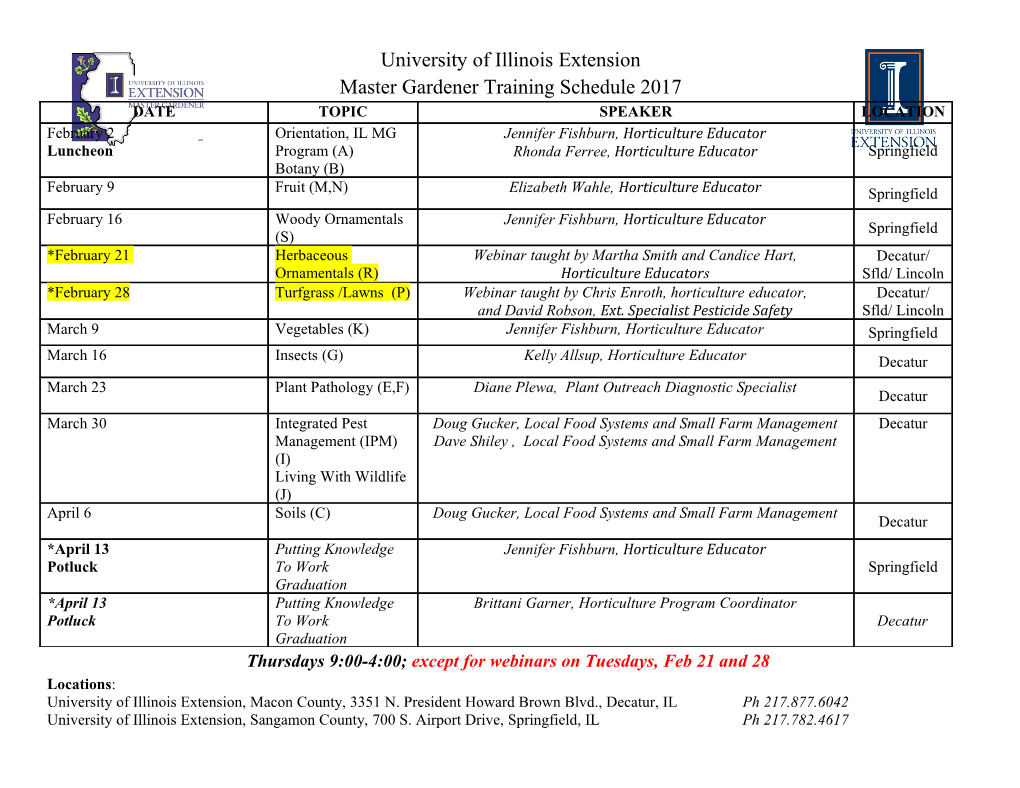
Homework 4 Solutions p Problem 5.1.4. LetpR = fm + n 2 j m; n 2 Zg. 2 2 (a) Show that m +pn 2 is a unit in R if and only if m − 2n = ±1. (b) Show that 1 + 2 has infinite order in R×. (c) Show that 1 and −1 are the only units that have finite order in R×. p Proof. (a) Define a map N : R ! Z where N(r) = m2 − 2n2 where r = m + n 2. One can verify that N is multiplicative, i.e. N(rs) = N(r)N(s) for r; s 2 R. Thus if r 2 R× then rs = q for some s 2 R. This implies 2 2 that N(r)N(s) = N(rs) = N(1) = 1 andp hence N(rp);N(s) 2 Zpimplies that m − 2n = N(r) = ±p1. 2 2 2 2 × For the converse observep that (m + n 2)(m +pn 2)(m − n 2) = (m − 2np) = 1, i.e. m + n 2 2 R . (b) Note that 1 + 2 > 1 and therefore (1 + 2)k > 1k = 1. Therefore 1 + 2 has infinite order. (c) Since R is a subring of R and the only elements in R with finite order in R× are ±1 it follows that the only elements in R× with finite order are ±1. Problem 5.1.5. Let R be a subset of an integral domain D. Prove that if R 6= f0g is a ring under the operations of D, then R is a subring of D. Proof. Since R is a ring under the operations of D, to show that R is a subring of D it's required to show that the identity 1R or R is the same as the identity 1D of D. Note that R and D have the same additive identity since 0R +0R = 0R = 0R +0D ) 0R = 0 = 0D. Now observe that 1R(1D −1R) = 1R ·1D −1R ·1R = 1R −1R = 0. Since D is an integral domain this implies that 1R = 0 or 1D − 1R = 0 and hence 1D = 1R since R 6= f0g ) 1R 6= 0. A counter example when D is not an integral domain is given by D = Z6 and R = f[0]6; [2]6; [4]6g as [4]6 is the identity of R while [1]6 is the identity of D. Problem 5.2.2. Let F be a field and let φ : F ! E be a ring homomorphism. Show that φ is either zero or one-to-one. Proof. If E is the zero ring then φ is zero. If E is not the zero ring then if x 2 F is nonzero then x−1 2 F and φ(x) · φ(x−1) = φ(x · x−1) = φ(1) = 1 6= 0. In particular φ(x) 6= 0. Thus ker φ = f0g and φ is one-to-one. Problem 5.2.5. Show that the identity mapping is the only ring homomorphism from Z into Z. Proof. The identity mapping 1Z is clearly a ring homomorphism. Moreover if φ : Z ! Z is a ring homomorphism then φ(n) = n for any n 2 Z since φ(0) = 0; φ(1) = 1 and for any n > 0; φ(n) = φ(1+···+1) = φ(1)+···+φ(1) = 1 + ··· + 1 = n and φ(−n) = −φ(n) = −n, i.e. φ = 1Z. Problem 5.2.21. Let R1;R2;:::;Rn be commutative rings. Complete the proof of Proposition 5.2.8, to show × ∼ × × × that R = R1 ⊕ R2 ⊕ · · · ⊕ Rn is a commutative ring. Then show that R = R1 × R2 × · · · × Rn . Proof. Since the multiplication on each component is associative and commutative it follows that the multiplica- tion on R is associative and commutative. The identity of R is given by (1; 1;:::; 1). Since the distributive law holds on the components it follows that the distributive law holds for R. Thus R is a commutative ring. Since (a1; a2; : : : ; an)(b1; b2; : : : ; bn) = (1; 1;:::; 1) if and only if aibi = 1 for all i. Thus (a1; a2; : : : ; an) 2 × × × × × × R () ai 2 Ri for all i, i.e. R = R1 × R2 × · · · × Rn . Problem 5.2.23. Show that if R is an integral domain with characteristic p > 0, then for all a; b 2 R we must n n n have (a + b)p = ap + bp. Show by induction that we must also have (a + b)p = ap + bp for all positive integers n. n n! Proof. Recall the binomial coefficient ( m ) = (n−m)!m! for 0 ≤ m ≤ n. Note that for p prime p j p! while p p - (p − m)! and p - m! for 0 < m < p. Thus p j ( m ) for 0 < m < n. By the binomial theorem we have X p (a + b)p = ap + bp + ambp−m m 0<m<p Since R has prime characteristic p it follows from the remarks above that (a + b)p = ap + bp. 1 n n n n+1 n Now suppose that (a + b)p = ap + bp for some positive integer n. Since (a + b)p = ((a + b)p )p, by the n+1 n n n+1 n+1 induction hypothesis and the base case it follows that (a + b)p = (ap + bp )p = ap + bp . Hence the induction is complete and the Freshman's dream is proven in this case. Problem 5.3.10. Let P be a prime ideal of the commutative ring R. Prove that if I and J are ideals of R and if I \ J ⊆ P , then either I ⊆ P or J ⊆ P . Proof. Suppose for a contradiction that I 6⊆ P and J 6⊆ P then there exists i 2 InP and j 2 JnP . However ij 2 I \ J since I and J are ideals. Since I \ J ⊆ P this implies that ij 2 P which contradicts P a prime ideal. Therefore I ⊆ P or J ⊆ P . Problem 5.3.12. Recall that an element of a commutative ring is said to be nilpotent if an = 0 for some positive integer n. (a) Show that the set N of all nilpotent elements of a commutative ring forms an ideal of a ring. (b) Show that R=N has no nonzero nilpotent elements. (c) Show that N ⊆ P for each prime ideal P of R. Proof. (a) First N is nonempty as 0 2 N. If a 2 N then an = 0 for some positive integer n and hence if r 2 R then (ra)n = rnan = 0. Now suppose a; b 2 N. then there are positive integers n and m such that an = bm = 0. Consider ajbn+m−j, if j ≥ n then aj = 0 so ajbn+m−j = 0 while if 0 ≤ j < n then bn+m−j = 0 so ajbn+m−j = 0. Hence by the binomial theorem it follows that (a + b)n+m = 0 so a + b 2 N. Thus N is an ideal. (b) Suppose r + N 2 R=N is nilpotent then (r + N)n = rn + N = 0 + N for some positive integer n. Hence rn 2 N implies that (rn)m = 0 for some positive integer m and hence rnm = 0, i.e. r 2 N. Thus r + N = 0 + N, so R=N has no nonzero nilpotent elements. (c) For a given a 2 N, an = 0 2 P for some positive integer n. Since P is a prime ideal it follows that a 2 P and hence N ⊆ P for any prime ideal P . Problem 5.3.18. Let R be a commutative ring with ideals I;J such that I ⊆ J ⊆ R. (a) Show that J=I is an ideal of R=I. (b) Show that the factor ring (R=I)=(J=I) is isomorphic to R=J. (c) Show that J=I is a prime (or maximal) ideal of R=I if and only if J is a prime (or maximal ideal) of R. Proof. (a) If π : R ! R=I is the natural projection then by Proposition 5.3.7 π(J) = J=I is an ideal of R=J. (b) Define f : R=I ! R=J by f(r + I) = r + J. Note that this is well defined since if a + I = b + I then a − b 2 I ⊆ J so a + J = b + J. The map f is clearly a surjective ring homomorphism and ker f = fr + I 2 R=I j r + J = 0 + Jg = fr + I 2 R=I j r 2 Jg = J=I. Thus by the fundamental homomorphism theorem (R=I)=(J=I) =∼ R=J. (c) Note that J is prime if and only if R=J is an integral domain. By part (b) we have R=J =∼ (R=I)=(J=I) and hence J is a prime ideal if and only if J=I is a prime ideal. The ideal J is maximal if and only if R=J =∼ (R=I)=(J=I) is a field if and only if J=I is a maximal ideal. Problem 5.3.19. Determine all prime ideals and maximal ideals of Zn. Proof. Recall that the ideals of Z are given by mZ and Z=mZ is an integral domain if and only if m is prime. Thus the prime ideals of Z are the ideals pZ for p prime and an ideal mZ contains nZ if and only if m j n. By the previous exercise the prime ideals of Z=nZ are pZ=nZ for p a prime factor of n. Note that Z=mZ is a field if and only if m is prime and hence the prime ideals and maximal ideals of Z coincide and hence the maximal and prime ideals of Zn coincide.
Details
-
File Typepdf
-
Upload Time-
-
Content LanguagesEnglish
-
Upload UserAnonymous/Not logged-in
-
File Pages2 Page
-
File Size-