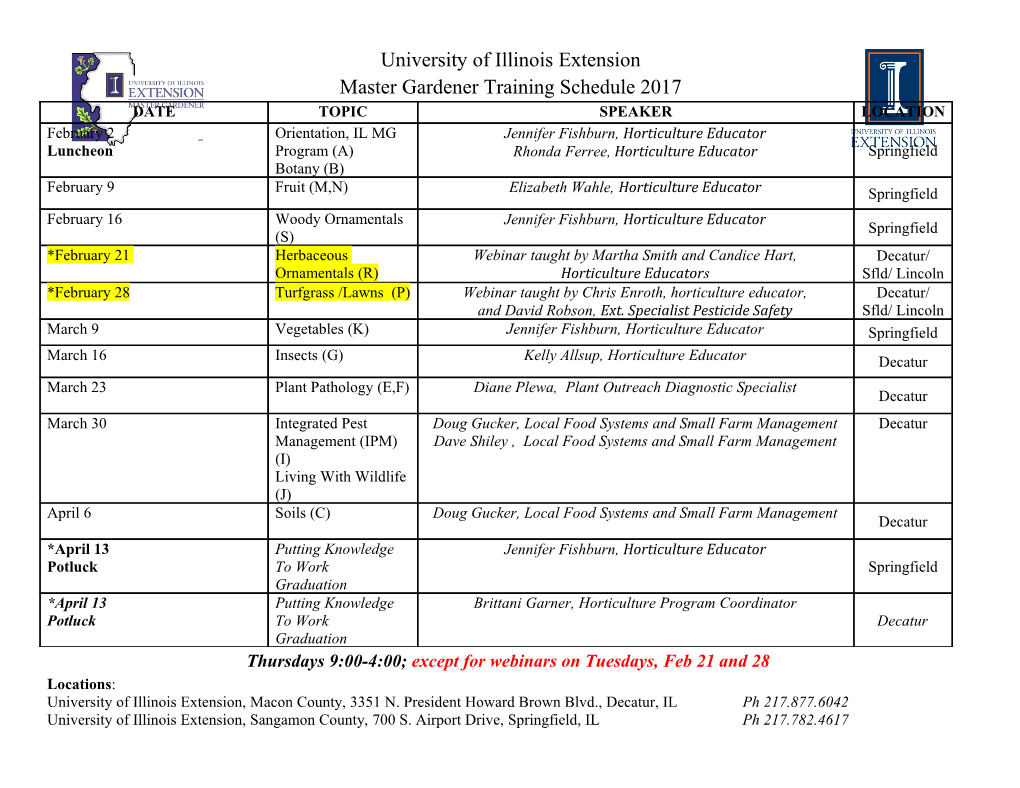
THEORY OF MEASURE AND INTEGRATION TOMASZ KOMOROWSKI 1. Abstract theory of measure and integration 1.1. Notions of σ-algebra and measurability. Suppose that X is a certain set. A family A of subsets of X is called a σ-algebra if i) ; 2 A, ii) if A 2 A then Ac := X n A 2 A, S+1 iii) if A1;A2;::: 2 A then i=1 Ai 2 A. Example 1. The family P(X) of all subsets of a given set X. Example 2. Suppose that A1;A2;::: is a partition of a set X, i.e. Ai \ Aj = ; if S+1 S i 6= i and j=1 Aj = X. Denote by A the family of all sets of the form j2Z Aj, where Z ⊂ f1; 2;:::g. Prove that A forms a σ-algebra. Example 3. Suppose that X is a topological space. Denote by T the family of all open subsets (topology). Then, introduce \ B(X) := A; where the intersection is over all σ-algebras A containing T . It is a σ-algebra and called the Borel σ-algebra. A pair (X; A) is called a measurable space. Any subset A of X that belongs to A is called measurable. A function f : X ! R¯ := R [ {−∞; +1g is measurable if [f(x) < a] belongs to A for any a 2 R. 1 2 TOMASZ KOMOROWSKI Conventions concerning symbols +1 and −∞. The following conventions shall be used throughout this lecture (1.1) 0 · 1 = 1 · 0 = 0; (1.2) x + 1 = +1; 8 x 2 R (1.3) x − 1 = −∞; 8 x 2 R x · (+1) = +1; 8 x 2 (0; +1)(1.4) x · (−∞) = −∞; 8 x 2 (0; +1)(1.5) x · (+1) = −∞; 8 x 2 (−∞; 0)(1.6) x · (−∞) = +1; 8 x 2 (−∞; −)(1.7) (1.8) ±∞ · ±∞ = +1; ±∞ · ∓∞ = −∞: Warning: The operation +1 − 1 is not defined! Exercise 1. Suppose that f; g : X ! R are measurable then 1)[ f(x)(<) ≤ a], [f(x) > (≥)a] are measurable, 2)[ f(x) < g(x)], [f(x) ≤ g(x)], [f(x) = g(x)] are measurable, 3) f + g, fg are measurable, 4) if g 6= 0 then f=g is measurable, 5) if a 2 R then af is measurable. ¯ Exercise 2. Suppose that fn : X ! R are measurable for any n ≥ 1, then 1) infffn; n ≥ 1g is measurable, 2) supffn; n ≥ 1g is measurable, 3) lim infn!+1 fn and lim supn!+1 fn are measurable, 4) the set Z on which limn!+1 fn exists is measurable, 5) if Z = X then limn!+1 fn is measurable. A function ( 1; x 2 A; 1 (x) = A 0; x 62 A: is called a characteristic function of A. Proposition 1.1. A characteristic function of A is measurable iff A is measurable. MEASURE AND INTEGRATION 3 A function f : X ! R is called simple if there exist a finite number of values c1; : : : ; cN 2 R and a partition fA1;:::;AN g of X such that each Ai is measurable and N X f = ci1Ai : i=1 Denote by Π(X) the class of all simple functions on X and by Π+(X) the subclass of all non-negative simple functions. Proposition 1.2. Suppose that f : X ! [0; +1] is measurable. Then, there exists a sequence (fn) ⊂ Π+(X) such that fn ≤ fn+1, n ≥ 1 and f(x) = lim fn(x); for any x 2 X: n!+1 Proof. Fix n ≥ 1 and define i i + 1 A = x : ≤ f(x) < ; i = 0; : : : ; n2n − 1 n;i 2n 2n and An;n2n = [x : n ≤ f(x)] : Let n2n X i f (x) := 1 : n 2n An;i i=0 n It is clear that x 2 An;i is equivalent with x 2 An+1;2i [ An+1;2i+1 for i = 0; : : : ; n2 − 1. n When x 2 An+1;2i, i = 0; : : : ; n2 − 1 then fn(x) = fn+1(x). In the case when x 2 An+1;2i+1 we have fn(x) < fn+1(x). Condition x 2 An;n2n is equivalent with 2n+1(n+1) [ x 2 An+1;j: j=2n+1n Observe that according to the definition j f (x) = ≥ n = f (x); for x 2 A ; j = 2n+1n; : : : 2n+1(n + 1): n+1 2n+1 n n+1;j Therefore fn(x) = n ≤ fn+1(x) for x 2 An;n2n . Note that 1 jf(x) − f (x)j ≤ ; when f(x) < n: n 2n This allows us to conclude that lim fn(x) = f(x); when f(x) < +1: n!+1 4 TOMASZ KOMOROWSKI If, however f(x) = +1, then n ≤ fn(x); 8 n ≥ 1 and the conclusion of the proposition also holds. 1.2. Definition of a measure. We start with the following definition: Suppose that (X; A) is a measurable space. A function µ : A! [0; +1] is called a measure if i) µ(;) = 0, ii) if A1;A2;::: is a family of sets from A that are pairwise disjoint, i.e. Ai\Aj = ; for i 6= j then +1 ! +1 [ X µ Ai = µ(Ai): i=1 i=1 Terminology: 1) the triple (X; A; µ) is called a measure space, 2) when µ(X) < +1 the measure is called finite, otherwise it is infinite. A measure is called σ-finite, when either it is finite, or in case it is inifnite, there exists a family X1 ⊂ X2 ⊂ ::: of measurable subsets such that +1 [ X = Xi i=1 and µ(Xi) < +1 for all i = 1; 2;::: 3) when µ(X) = 1 the measure is called a probability measure, the respective triple (X; A; µ) is called a probability space. 4) if the target set [0; +1] in the definition of a measure is replaced by (−∞; +1], or [−∞; +1) then µ is called a signed measure (a σ-additive set function or charged measure, or simply a charge). Example 1. Suppose that X0 is a subset of X. Let A := P(X). Define a measure: µ(A) := card(A \ X0);A 2 P(X); where card(A) stands for the cardinality of set A. A set A 2 A is called an atom for a measure µ if µ(A) > 0 and for any A1;A2 2 A such that A = A1 [ A2 and A1 \ A2 = ; we have either µ(A1) = 0, or µ(A2) = 0. MEASURE AND INTEGRATION 5 Note that any point from X0 in case of the measure defined in Example 1 is an atom of mass 1. In the particular case X0 = fx0g the respective measure is denoted by δx0 and it is called Dirac's delta measure. It can be written as δx0 (A) = 1A(x0); 8 A 2 P(X): Example 2. Suppose that X0 is countable and f : X0 ! [0; +1]. Define a measure: X µ(A) := f(x);A 2 P(X): x2A\X0 Example 3. Suppose that A1;A2;::: is a partition of a set X and A is the σ-algebra S of the unions j2Z Aj; where Z ⊂ f1; 2;:::g. Suppose that f : N ! [0; +1]. Let ! [ X µ Aj := f(j): j2Z j2Z It is a measure. A measure µ is called atomless if it has no atoms. 1.3. Some elementary properties of a measure. The following properties of measures shall be useful in the sequel. Proposition 1.3. Suppose that (X; A; µ) is a measure space. Then, 1) if A; B 2 A and B ⊂ A then, µ(A) ≥ µ(B), S+1 2) if A = n=1 An and A1 ⊂ A2 ⊂ ::: then, µ(A) = lim µ(An); n!+1 T+1 3) if A = n=1 An and A1 ⊃ A2 ⊃ ::: and µ(A1) < +1 then, µ(A) = lim µ(An); n!+1 4) if A1;A2;::: is a family of sets from A then, +1 ! +1 [ X µ Ai ≤ µ(Ai): i=1 i=1 Proof. Part 1). Note that A = B [ (A n B), where B and A n B are disjoint. Therefore µ(A) = µ(B [ (A n B)) = µ(B) + µ(A n B) ≥ µ(B): 6 TOMASZ KOMOROWSKI Part 2). Note that +1 [ A = Bn; n=1 where B1 = A1 and Bn+1 := An+1 n An; 8 n ≥ 1: Note that (Bn) are pairwise disjoint and n [ (1.9) An = Bj; j=1 and +1 [ (1.10) A = Bj: j=1 Therefore n X µ(An) = µ(Bj): j=1 and +1 n X X µ(A) = µ(Bj) = lim µ(Bj) = lim µ(An): n!+1 n!+1 j=1 j=1 Part 3). Note that +1 [ A1 n A = (A1 n An) n=1 and A1 n A1 ⊂ (A1 n A2) ⊂ :::. From the (proven) part 1) we have (1.11) µ(A1 n A) = lim µ(A1 n An): n!+1 However, since for each n ≥ 1 we have A1 = (A1 n An) [ An and (A1 n An) \ An = ; we can write µ(A1) = µ(A1 n An) + µ(An) and because µ(An) < +1 we have µ(A1 n An) = µ(A1) − µ(An): By the same token µ(A1 n A) = µ(A1) − µ(A): Combining the above with (1.11) we get µ(A1) − µ(A) = µ(A1) − lim µ(An) n!+1 MEASURE AND INTEGRATION 7 and the conclusion of this part follows because µ(A1) < +1. Part 4). Let +1 [ A := Ai: i=1 Note that +1 [ A = Bi; i=1 where B1 := A1;Bn := An n (A1 [ ::: [ An−1); n ≥ 2: Since the sets (Bn) are pairwise disjoint and Bn ⊂ An we can write +1 +1 X X µ(A) = µ(Bi) ≤ µ(Ai): i=1 i=1 Example 1.
Details
-
File Typepdf
-
Upload Time-
-
Content LanguagesEnglish
-
Upload UserAnonymous/Not logged-in
-
File Pages88 Page
-
File Size-