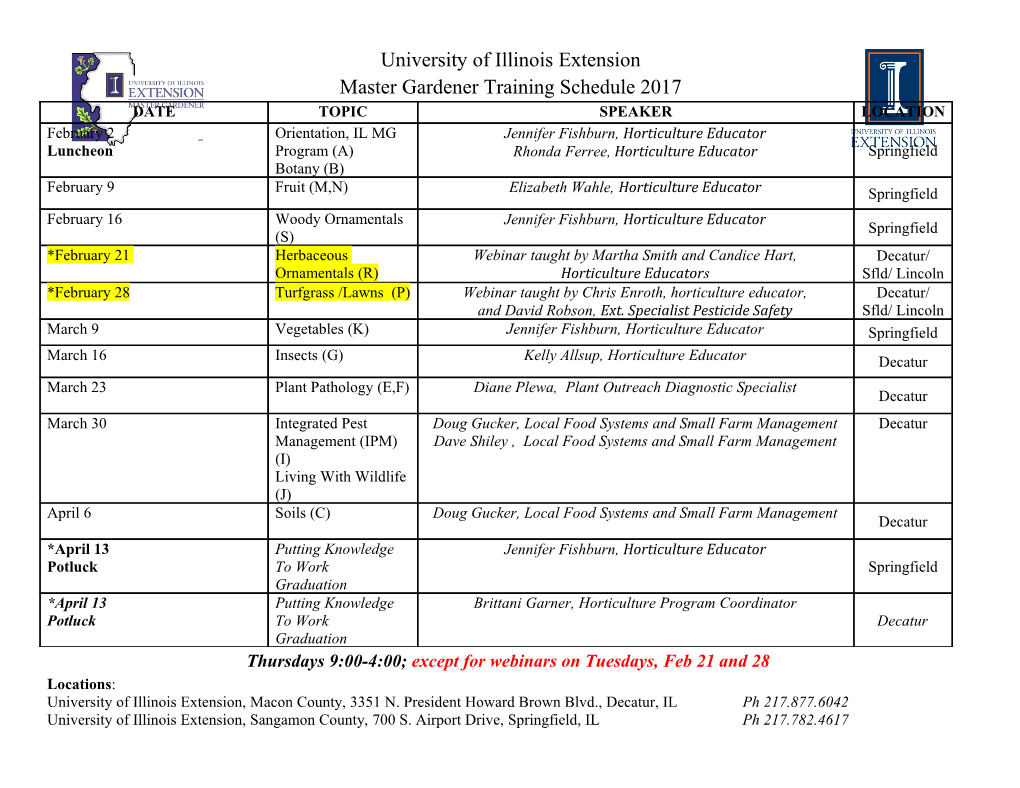
SPRAY FORMING OF Tl-ALUMINIDE-BASED ALLOYS J. W. Sears**, G. ltoh*,'and M. H. Loretto* *IRC in Materials for High Performance Applications The University of Birmingham, Edgbaston, England B15 2TI, **ManLabs, A Division of Alcan Aluminium Corporation 21 Erie Street, Cambridge, Massachusetts, 02139 ABSTRACT The aim of this programme is to spray form Ti Aluminides free from porosity and interstitial contaminatio.n. Initial work wa-s carried out by atomising IMI-318 (Ti-6-4) and Ti-aluminide alloys to assess the operation of the cold-wall induction bottom-pour (CWIBP) as a clean melting system. Ti-aluminide-based alloys have been sprayed onto flat and tubular substrates. The IRC plasma melting system at The University of Birmingham, Edgbaston, England, was used to provide ingot feed stock for the spray deposition and atomisation. The plasma system, used for casting ingots, was able to be converted for atomisation or spray deposition. Spray forming was facilitated by the use of a scanning or centrifugal atomiser. The operation of this process will be discussed and the effects of various operating parameters on the spray deposit product will be presented. The chemistry, microstructure, homogeneity and macrostructure of the as sprayed material has been examined. INTRODUCTION The Ti aluminides form a technologically important group of intermetallic compounds. TiAI (y) is one of these which exhibits great promise for commercial exploitation, being strong at elevated temperatures while also exhibiting superior oxidation resistance. However, the compound is brittle at ambient temperatures and this limits its application. Possible sources of this brittleness include compound purity, planarity of slip and the nucleation of voids at the points of intersection of deformation twins. Some attempts have been made to improve the room temperature ductility by alloying additions, the introduction of a dispersion of hard second phases, and the control of grain size. Consequently, there has been considerable interest in the application of powder metallurgy (PM) and rapid solidification processing (RSP) to the preparation of these materials. Research has been focused on IM route by isothermal forging and the powder metallurgy (PM) approach through powder consolidation via HIP, plasma spraying and mechanical alloying. Although, there has been considerable success in the IM and PM techniques, they have proved to be very expensive'. The spray forming of a deposit directly from the melt offers a more economical process to produce such difficult to form materials. As will be shown, deposits of Ti-aluminides can be produced directly from a stream of molten metal without the handling problems associated with the P M route. Tilanium '92 Science and Technology Edited by F.H. Froes and I. Caplan The Minerals, Melals & Materials Society, 1993 987 It has been pointed out that there are as many as five different solid phases possible for an alloy of composition Ti-52AI which has been undercooled -200 K below the equilibrium liquidus, these being ~(bee), a (hep), and the intermetallic compounds y (Llo), B2, and a2 (D019)2. It is known that when processing with the cold-wall induction bottom-pour (CWIBP), described below, that C and 02 can be introduced into the powders. Carbon is highly insoluble in TiAl, and the compound Ti2AIC may precipitate during consolidation and/or heat-treatment of the powder3. Part of the investigation, involving the powders and spray deposits, will be the examination of Ti-alloy particles before and after heat treatment to determine the extent of the C contamination. Oxygen affects the beta transus temperature of these alloys and adds to the material inherent brittleness. COLD-WALL INDUCTION BOTTOM-POUR (CWIBP) The CWIBP system includes a melt chamber capable of being pressurised to 170 kPa. This allows for the flexibility to ramp an overpressure in the melt chamber to account for the change in metalistatic head during the metal pour. A controlled overpressure allows for a constant metal flow rate which is critical in producing uniform spray deposits. The cold-wall crucible is a segmented, water-cooled, induction heated device. An induction-heated graphite nozzle at the base of this crucible constricts the molten metal into a stream for atomisation and spray forming. Figure 1: a) CWIBP crucible installed in the IRC PACH furnace. b) Schematic of the CWIBP system. Plasma-arc cold-hearth (PACH) melted ingots of IMl-318 (Ti-6-4), Ti-48AI, Ti-52Al, Ti- 48Al-2Nb-2Mn and Super-a2 (Ti-25Al-10Nb-3V-1Mo) were produced, as discussed before4, to provide billet material for subsequent thermal processing, powder production and consolidation and spray-formed deposits. Powders of IMl-318 (Ti-6-4), Ti-52AI and Super-a2 have been produced by CWIBP. The Ti-aluminide alloys, Ti-48AI, Ti-52AI, Ti-48Al-2Nb-2Mn and Super-a2 have been spray formed onto sheet and tubes. The spray system utilises the same CWIBP crucible that was used in powder production to provide a controlled, molten stream of metal to a scanning atomiser for the production of flat or drum preforms or to a centrifugal atomiser (CSD) for the production of rings. A photograph and schematic of the CWIPB device is shown in Figure l(a) and (b). The operation of this process has been discussed before5. The effects of various operating parameters on the spray deposit product will be presented. The chemistry, homogeneity and microstructure of the as sprayed material has been examined and compared with material made through both the PM and JM routes. The plasma-melted, as-cast and heat-treated microstructures of Ti aluminides have been described elsewhere6,7. ATOMISATION Alloys of IMI-318 (Ti-6-4), y-Ti (Ti-52Al) and Super a2-Ti were atomised. CWJBP served as the melt source to provide molten metal to the atomisation die for these alloys. Metal 988 temperature was measured using an IRCON, two-colour pyrometer. Superheat of - 30°C was obtained when melting IMI-318 (Ti-6-4) and ... 20°C for most of the Ti-aluminides. When the charge is completely melted, the nozzle is heated and molten metal begins to flow when the metal directly above the nozzle melts. Low powder yields, due to nozzle blow back and stream instability, were experienced during these early runs with lots of 3 to 6 kgs being produced from 25 kg charges (6 to 8 kgs retained in skull). Metal to gas flow rates of about 1:1 were planned. The details of the atomisation system has been described in detail before3. Shown in Table 1 is the data from the first atomisation runs. Mean particle sizes for these runs were higher than expected. Based on the data collected from these runs, it was found that the over pressure compensation was incorrect, resulting in higher than expected metal flow and therefore, low gas to metal flow rates resulting in larger powder particle size. Nozzle blockage by metal blow back was a main contributor to low yields. It is anticipated that, a change to an lower nozzle angle (<15°) will correct the blow back problem. Table 1. Operating conditions from the initial atomisation runs. RUN ALLOY CHARGE SKULL GAS/MET YIELD MEAN NOZZLE JET # WEIGHT WEIGHT RATIO• %*• SIZE® DIAMETER ANGLE l IMl-318 25 kgs 8.1 kgs 0.72 5.3 123 µm 5.0 mm 7.5° 2 IMl-318 24 kgs 7.9 kgs 0.41 2.0 133 µm 4.0 mm JOO 4 Ti-52AI 14 kgs 4.5 kgs 0.86 41.6 158 µm 5.0 mm 15° 5 Super-a2 27 kgs 7.2 kgs 0.62 9.3 144 µm 5.0 mm 15° •Gas I Met Ratio= kgs min-I Ar Gas+ kgs min-I Metal, ••Yield%= Total weight powder< 250 µm produced + Weight of metal poured through atomiser, @Mean size is at the 50% cumulative weight for all powders < 250 µm. IMI-318 <Ti-6-4) Powder The powder particles shown in Figure 2(a) are representative of the material produced by this process. Initial chemistry results on IMI-318, Ti-52AI and Super-a2 powder are shown in Table 2. Analysis was performed on several size fractions of powder with the larger size fractions consistently having less C and 02 contamination. Results on IMI-318 powders indicated C levels from 0.06 to 0.08 wt.%,. This indicates that C pickup is from 0.04 to 0.06 wt.%, with 65 µm powder about an 0.06 wt.% increase and 150 µm powder an 0.04 wt.% increase. In the main, the increase in C contamination in the particles is most likely due to graphite nozzle erosion. Upon examination after these runs, it was found that some C-based insulating material broke-down as a result of the nozzle blockage. A C-based film was discovered coating the chamber walls, and would have coated the powders also. This would explain why the 65 µm particles have a higher concentration of C than the 150 µm powder, owing to the fact that smaller particles have a higher surface to volume ratio. For a similar reason, smaller particle have higher 02 levels due to surface oxides. Table 2: Ti Alloy Powder 02 and C Levels from the Initial Atomisation Runs as a function of particle size. RUN # ALLOY 45-90 µm 125-180 µm 45-90 µm 125-180 µm Oxv2en ppm Oxv2en ppm Carbon wt% Carbon wt% 1 IMl-318 2900 2600 0.08 0.06 2 IMl-318 4100 2500 0.08 0.06 4 Ti-52AI 1050 900 0.02 O.Dl 5 Super-a2 1850 1000 0.09 0.04 A TEM micrograph of IMl-318, ... 50 µm powder, Figure 2(b), shows a martensitic structure, common in rapidly solidified conventional Ti alloys.
Details
-
File Typepdf
-
Upload Time-
-
Content LanguagesEnglish
-
Upload UserAnonymous/Not logged-in
-
File Pages8 Page
-
File Size-