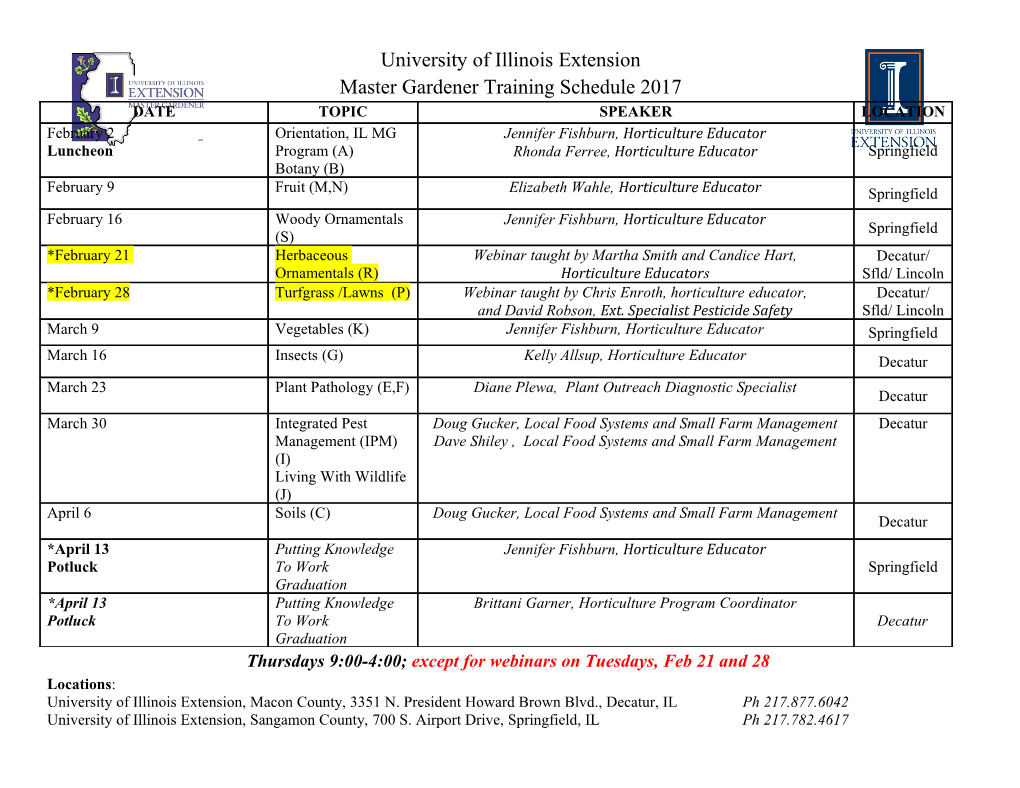
SOLA, 2010, Vol. 6A, 025−028, doi:10.2151/sola.6A-007 25 Characteristics of Supercells in the Rainband of Numerically Simulated Cyclone Sidr Nasreen Akter1 and Kazuhisa Tsuboki1, 2 1Hydrospheric Atmospheric Research Center, Nagoya University, Nagoya, Japan 2Frontier Research Center for Global Change, JAMSTEC, Yokohama, Japan (Figs. 1b and 1c). The cell indicated in Fig. 1b is ~116 km away Abstract from the radar, and its local altitude is ~1 km. Also, no sounding data are available. The observed data are not sufficient for inves- Cyclone Sidr had an intense rainband east of the cyclone tigation of the detailed structure and intensity of the convective center. The rainband exhibited two strong convective lines (band cells along the band. axes) composed of convective cells. To study the characteristics of Therefore, the present study aims to clarify the characteristics the convective cells, a simulation was performed at 1-km resolu- of the convective cells in the rainband of Cyclone Sidr over the tion by using a cloud-resolving model. In both band axes, some sea and the environmental factors affecting cell development. For cells showed the characteristic structure of a supercell. Supercells this purpose, a high-resolution simulation was performed using within the outer axis had stronger updraft, more intense precipita- the Cloud-Resolving Storm Simulator (CReSS) model (Tsuboki tion, and a longer lifetime than those of the inner axis. The values and Sakakibara 2007). of the CAPE and helicity are different in the strong vertical shear environment of the inner and outer axes. A large moisture flux was present throughout the troposphere on the east side of the outer 2. Numerical simulation and verification axis. On the other hand, the upper level west of the inner axis was relatively dry. These are additional factors affecting the differ- The high-resolution simulation was carried out using a ences in cell characteristics in the two band axes. horizontal grid spacing of 1 km. The horizontal grid number was 903 × 1206. The computational domain was 85.69°E−94.78°E 1. Introduction Cyclone Sidr is a category-4-equivalent cyclone of the Indian Ocean, which originated in the Bay of Bengal and made landfall over Bangladesh on November 15, 2007. It had a prominent rainband east of the center, which was associated with heavy rain- fall and severe flooding as well as damaging winds. The intense precipitation of the rainband was determined by its constituent convective cells. Previous studies have found supercells in tropical cyclone rainbands (Spratt et al. 1997; Lee et al. 2008; Eastin and Link 2009). They have a deep, continuously rotating updraft that is not interrupted by a downdraft. Supercells found in the rainband are mostly the mini-supercell type. Mini-supercells are smaller and short-lived, having shallow mesocyclones (MCs) (Burgess et al. 1995; Suzuki et al. 2000), in contrast to typical midlatitude super- cells (Moller et al. 1994). They are characterized by maximum reflectivities of less than 50−55 dBZ and echo tops < 10 km (NWS 2004). McCaul and Weisman (1996) proposed that in a hurricane environment, weak low-level cold pools restrict surface vorticity development, resulting in shallow supercells. It is evident from the literature that environmental characteristics, such as strong low-level wind shear, moderate storm-relative environmental he- licity (SREH), and moderate to large CAPE, are favorable for the development of supercells in the rainband at the time of landfall or over land (McCaul 1991; McCaul and Weisman 1996; Baker et al. 2009). McCaul and Weisman (2001) suggested that the CAPE and shear are important determinants of the morphology and intensity of convective cells. However, studies of the characteristics of cells in the rainbands and their environment over the ocean are still limited. Moreover, during the approach of Cyclone Sidr, only a single Doppler radar at a range of 440 km was operating over the entire coast. Its horizontal resolution in Doppler mode is 625 m, and the azimuthal resolution is roughly 2.5 km. Supercell-like structures (weak hook-shaped echoes and positive-negative velocity cou- plets) are observed with much data noise in the velocity signature Fig. 1. (a) Plan position indicator (PPI) display of radar reflectivity at 0° elevation for 1000 UTC November 15, 2007 with the observed track (solid red line). (b) Reflectivity and (c) Doppler velocity, respectively, indicated in (a). (d) Simulated rain rate (shading) at 1000 UTC and track (solid red Corresponding author: Nasreen Akter, Hydrospheric Atmospheric Research −2 −1 Center, Nagoya University, Nagoya 464-8601, Japan. E-mail: akter@rain. line). Arrows represent surface moisture flux vectors (kg m s ). Dashed hyarc.nagoya-u.ac.jp. ©2010, the Meteorological Society of Japan. lines indicate the two band axes. Oval shows the north cluster. 26 Akter and Tsuboki, Characteristics of Supercells in Cyclone Sidr and 13.97°N−25.05°N. The height of the domain was 30 km with into the north cluster (Fig. 1d). For this purpose, three isolated stretched grid spacing from 100 m at the surface to 564 m at the convective cells of the inner axis (a, b, and c) and three of the top level. The cloud microphysical process used to predict the outer axis (d, e, and f) were selected. Cells a, d, and e are marked mixing ratios of cloud water, rain, cloud ice, snow, and graupel in Fig 1d. The results of an inner-axis cell (a) and an outer-axis and the number densities of cloud, ice, snow, and graupel was the cell (d) are presented in detail as representative cells. The proper- bulk cold rain type. The subgrid-scale turbulence was parameter- ties of other cells are summarized later. ized using 1.5-order closure with turbulent kinetic energy. The The time sequences of cells a and d are shown in Fig. 2a. Cell radiation process was considered in calculating the heat balance of d is more intense and larger than cell a. Figures 2b and 2c show the ground surface. The references for the physical parameterized the vertical component of vorticity at 0950 UTC for cell a (cross schemes we used were cited in Tsuboki and Sakakibara (2007). section at 90.17°E) and cell d (at 90.58°E), respectively. In both The initial and boundary conditions were provided by the six- cases, positive and negative vorticities are present in the cells. hourly data of the Japan Meteorological Agency-Global Spectrum Both cells have strong positive vorticity on the east side and weak Model (JMA-GSM) output. The JMA-Merged satellite and in-situ negative vorticity on the west. A strong updraft is associated with data Global Daily Sea Surface Temperature (MGDSST) data were the positive vorticity, and a weak downdraft is associated with the used for the lower boundary condition in the ocean region. The negative vorticity. Inner-axis cell a has a weaker vortex pair and period of the simulation experiment was 24 h, from 0000 UTC weaker vertical velocity than outer-axis cell d. November 15 to 0000 UTC November 16, 2007. As indicated in Figs. 3a and 3b, the mixing ratio of precipita- Cyclone Sidr was simulated well in the experiment. The result tion (sum of rain, snow, and graupel) of cell a is ~9 g kg−1 and is verified by radar data. Figures 1a and 1d show the observed extends up to ~8 km in height. The downdraft is very weak (~ and simulated rainfall distributions, respectively, at 1000 UTC −1 m s−1). In cell d (Figs. 3c and 3d), the horizontal precipitation November 15, along with the cyclone track. Both the simulated structure shows a bean-shaped pattern with the intense updraft and and observed rainbands exhibit two band axes (strong convection vorticity of an MC. The strong updraft results in a large mixing lines). The outer axis contains isolated cells, and the inner axis is ratio of precipitation (> 10 g kg−1), which extends up to ~16 km. mostly composed of isolated cells as well as cluster or line types The downdraft is ~−5 m s−1 in the cell. In both cases, the updraft of convection. The radar reflectivity image (Fig. 1a) shows that is separated from the downdraft, and cell-relative flows approach the rainfall intensity in the outer axis is greater than that in the cells from the east below 2 km in height. inner axis. Similar differences in convection intensity are also The characteristics of the six simulated cells are summarized found in the simulation (Fig. 1d). in Table 1. The outer-axis cells contain MCs at higher levels with vorticity > 0.014 s−1. The dimensions, updraft, downdraft, and pre- cipitation intensities are larger than those of inner-axis cells. The 3. Convective cells lifetime of a cell, which is defined as the period from formation to dissipation or merging into the north cluster, is ≥ 2 h for outer-axis Convective cells were formed and were arranged along low- cells, whereas it is less than 1.5 h for inner-axis cells. The heights level convergence lines. After forming, they moved north-north- of inner-axis cells are ≤ 10 km, whereas those of outer-axis cells westward. Some cells dissipated and others merged. To examine are ≥ 14 km. A boundary of 2 × 10−3 s−1 vorticity contours is the basic characteristics of the convective cells, we investigated considered to mark the height and diameter of an MC. Cell dimen- isolated cells from formation to dissipation or until they merged sions are confined to the precipitation mixing ratio of 2 g kg−1. Fig. 3. Cell a: (a) horizontal cross section of precipitation mixing ratio Fig.
Details
-
File Typepdf
-
Upload Time-
-
Content LanguagesEnglish
-
Upload UserAnonymous/Not logged-in
-
File Pages4 Page
-
File Size-