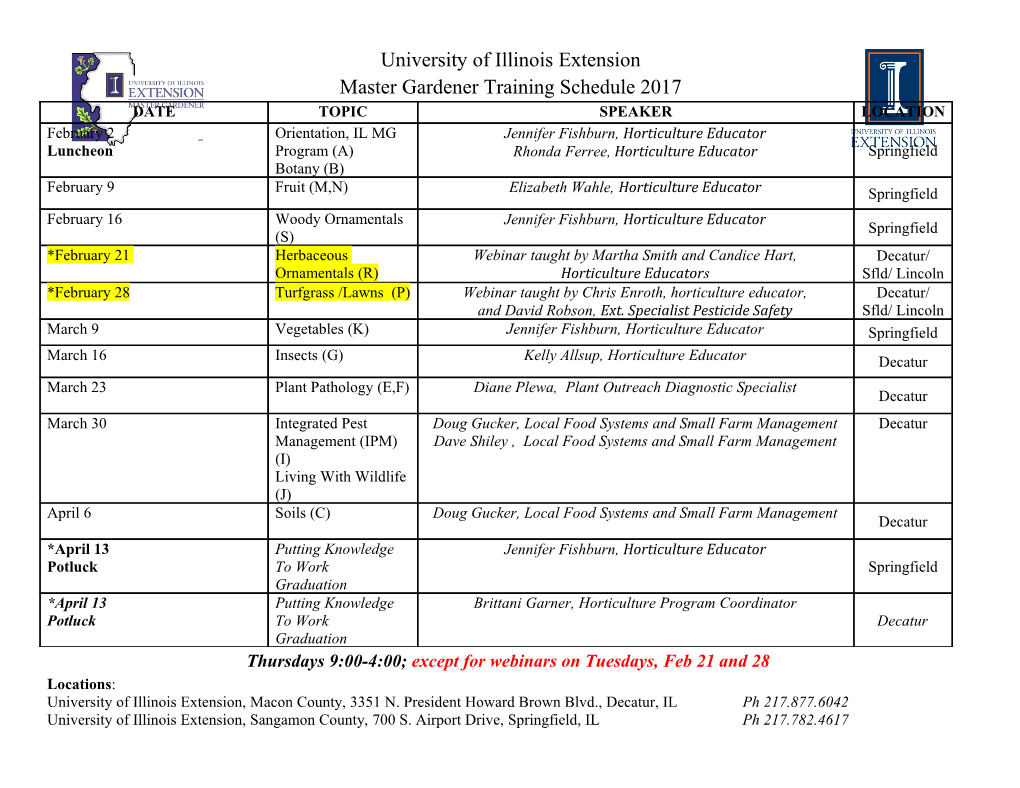
397 SOIL SALINITY CONTROL UNDER BARLEY CULTIVATION USING A LABORATORY DRY DRAINAGE MODEL Shahab Ansari 1,*, Behrouz Mostafazadeh-Fard 2, Jahangir Abedi Koupai3 Abstract The drainage of agricultural fields is carried out in order to control soil salinity and the water table. Conventional drainage methods such as lateral drainage and interceptor drains have been used for many years. These methods increase agriculture production; but they are expensive and often cause environmental contaminations. One of the inexpensive and more environmental friendly methods that can be used in arid and semi-arid regions to remove excess salts from irrigated lands to non- irrigated or fallow lands is dry drainage. In the dry drainage method, natural soil system and the evaporation of fallow land is used to control soil salinity and the water table of irrigated land. There are few studies about dry drainage concepts. it is also important to study soil salt changes over time because of salt movements from irrigated areas to non-irrigated areas especially under plant cultivation. In this study a laboratory model which is able to simulate dry drainage was used to investigate soil salts transport under barley cultivation. The model was studied during the barley growing season and for a constant water table. During the growing season soil salinities of irrigated and non-irrigated areas were measured at different time. The Results showed that dry drainage can control the soil salinity of an irrigated area. The excess salts leached from an irrigated area and accumulated in the non-irrigated area and the leaching rate changed over time. Soil surface salinities of non-irrigated areas increased with time. At the end of the experiment, the increase of the mean soil salinity of the non-irrigated area was 2.81 times more than the increase of the mean soil salinity of the irrigated area. In arid and semi-arid regions where suitable conditions exist, dry drainage can be used as a useful management tool to control soil salinity. KEY WORDS: Barley, Dry drainage, Salt movement, Shallow ground water. 1 Ph. D. Student, Water Engineering Department, College of Agriculture, Isfahan University of Technology, Isfahan, Iran. *Corresponding author: [email protected]; Isfahan University of Technology, Isfahan 84156-83111, Iran Tel: +989139780991. 2 Professor, Water Engineering Department, College of Agriculture, Isfahan University of Technology, Isfahan, Iran. Isfahan University of Technology, Isfahan 84156-83111, Iran. [email protected], Tel: +983133913430. 3 Professor, Water Engineering Department, College of Agriculture, Isfahan University of Technology, Isfahan, Iran. Isfahan University of Technology, Isfahan 84156-83111, Iran. [email protected], Tel: +983133913433. 398 Introduction During the last 3-4 decades, as the demand for agricultural productions increased the irrigated lands also increased up to 300%. This has imposed a further increase in soil salinization and a relative decrease in crop yield (Poustini and Siosemardeh, 2004). In arid and semi-arid regions, farmers often use saline water to irrigate their fields because of shortage of good quality water resources. This causes soil and water contaminations and reduces agricultural productions. Drainage is a common method to reduce salt accumulation in soil especially when irrigating with saline water. Salt leaching is one of the most important aims of the drainage systems, but it causes fertilities and pesticides to be removed from soil profile and pollute surface water and ground water resources (Deng, 1998). In recent years, researchers have tried to find more suitable and environmental acceptable method for drainage of agricultural fields. For example subsurface irrigation and controlled drainage are irrigation methods which can reduce pollution and still provide sufficient irrigation requirement (Ragab, 2002; Skaggs, 1999). Results of different researches have shown that it is beneficial if crop rotation is considered for land reclamation programs to decrease soil salinity. The subsurface drainage systems in agricultural fields are expensive and cause environmental pollutions. Dry drainage is a new concept for drainage of agricultural fields which has less environmental impacts and with low cost as compared to subsurface drainage systems can reduce soil salts and leach excess salts (Konukcu and et al., 2006). Greenwood et al. (1994) first have introduced dry drainage. In this method part of the field is considered as irrigated area and another part is considered as non-irrigated area or fallow land. Drainage occurred through soil of irrigated area and salts will leach to the non-irrigated area or fallow land. The gradients from irrigated area to non-irrigated area cause salts and excess water movements (Khouri, 1998). Dry drainage is efficient and applicable for conditions of high water table, high capillary rise and high potential of evaporation. These conditions are visible in many arid and semi-arid regions that suffer from salinity problems (Khouri, 1998). Konukcu, et al. (2006) reported that dry drainage is a sustainable solution to water logging and salinity problems in irrigated area. There are few researches about dry drainage and its practical use (JingWei and et al., 2009; Khouri, 1998). Previous studies had confirmed that dry drainage is more economical and environmental acceptable drainage method for agricultural fields. Therefore, the objective of this study was to simulate soil salinity under dry drainage with barley cultivation using a laboratory model. Materials and methods In this study a laboratory dry drainage simulation model was used to investigate soil salinity control under barley cultivation at Isfahan University of Technology, Isfahan, Iran. The model was made from galvanized sheet with thickness of 4 mm and with dimensions of 2 m length, 0.5 m width and 1 m height as shown in Fig.1. 399 0.5 m 1 m 0.5 m 0.5 m Fallow Irrigated Fallow area area area Soil surface 1 m Soil sluice Piezometer Soil sample collection 2 m Figure 1. The schematic of the laboratory dry drainage simulation model To observe water flow and ground water level in the model, one side of the model was made of the Plexiglas with thickness of 8 mm. Another side of the model had a grid system with vertical distance of 0.2 m and horizontal distance of 0.1 m. Half of these grid points were connected to polyethylene piezometers with inside diameter of 5 mm to measure and monitor groundwater level and other half were used to collect soil samples for soil moisture and soil salinity measurements. To drain water and to obtain desire water table, a drainage valve was installed at the bottom of the model. To obtain uniform water table, sand filter was placed at the bottom of the model. A water level controller connected to the drainage valve at the bottom of the model. It was able to raise water from the bottom to the top smoothly until it reaches the desire water level which was 0.6 m from the soil surface. The soil used for the study was loamy which was taken from an agricultural field. The physical and chemical characteristics of the soil are given in Table 1. The model was filled layer by layer by the soil and each layer was compacted uniformly to reach the bulk density which is close to bulk density of actual field. Model was divided into two equal sections of irrigated and non-irrigated (fallow) areas in such a way that due to hydraulic gradient changes, soil water and salt movements occur naturally between these two sections. The irrigated area was cultivated by barley and was irrigated with irrigation water which its characteristics are shown in Table 2. Irrigation water salinity was obtained by mixing good quality irrigation water with the saline water. The maximum irrigation interval was 3 days and irrigation amount changed with time. With irrigation, soil moisture was raised to field capacity and water table was raised to 0.6 m depth below soil surface. The experiment was carried out for one complete growth season of the barley 400 and mass soil water and salinity of irrigated area and non-irrigated area were measured at five different times of 0, 21, 42, 63 and 84 days after planting using soil samples taken at different locations of the model. Table 1. The characteristics of soil Sand Silt Clay Bulk density EC Characteristics pH (%) (gr/m3) (dS/m) value 52 28 20 1.6 3 8 Table 2. The characteristics of irrigation water Na+ Ca2+ Mg2+ K+ EC Characteristics pH (meq/L) (dS/m) value 5.99 9.15 8.14 2.94 3 7.5 Results and discussion Fig. 2 shows soil surface moistures of irrigated and non-irrigated areas for 0, 21, 42, 63 and 84 days after planting. Soil moistures of irrigated area and non-irrigated areas were equal at the beginning of the experiment. After plant cultivation, for first few weeks the difference between soil surface moistures increased due to irrigations. The differences decreased because of water movements from irrigated area to the non-irrigated area. Water movements occurred due to hydraulic gradient between two areas. Irrigations of irrigated area and also evaporation of soil surface of non-irrigated area increased the hydraulic gradient and caused water movements from irrigated area to non-irrigated area. Figure 2. Soil surface moistures for different times after planting Fig. 3 shows soil moistures at different depths for 84 days after planting for irrigated and non- irrigated areas. Soil moistures in both areas increased by soil depth due to capillary rise from water 401 table. Soil moisture at each depth for the irrigated area was more than the non-irrigated area. The difference of soil moisture at each depth of irrigated and non-irrigated areas increased as soil depth decreased due to irrigation of irrigated area and evaporation of non-irrigated area.
Details
-
File Typepdf
-
Upload Time-
-
Content LanguagesEnglish
-
Upload UserAnonymous/Not logged-in
-
File Pages210 Page
-
File Size-