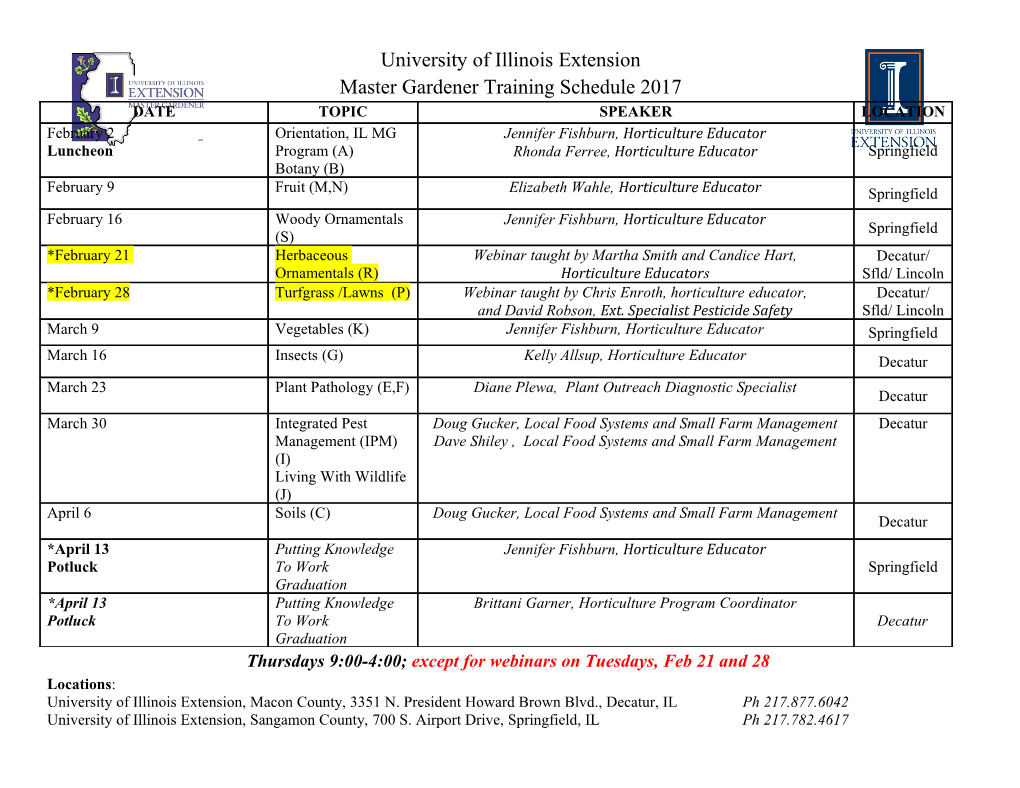
1 Splay-fault origin for the Yakima fold-and-thrust belt, Washington State 2 3 Thomas L. Pratt, United States Geological Survey, School of Oceanography, Box 357940, 4 University of Washington, Seattle, WA 98115 5 6 ABSTRACT 7 The Yakima fold-and-thrust belt (YFTB) is a set of anticlines above reverse faults in the 8 Miocene Columbia River Basalt (CRB) flows of Washington State. The YFTB is bisected by the 9 ~1100-km-long Olympic-Wallowa geomorphic lineament (OWL). There is considerable debate 10 about the origin and earthquake potential of the YFTB and OWL, which lie near six major dams 11 and a large nuclear waste storage site. Here I show that the trends of the YFTB anticlines relative 12 to the OWL match remarkably well the trends of the principal stresses determined from Linear 13 Elastic Fracture Mechanics (LEFM) modeling of the end of a vertical strike-slip fault. From this 14 comparison and the termination of some YFTB anticlines at the OWL, I argue that the YFTB 15 formed as splay faults caused by an abrupt decrease in the amount of strike-slip motion along the 16 OWL. If this hypothesis is correct, the OWL and YFTB are likely interconnected, deeply-rooted 17 structures capable of large earthquakes. 18 19 20 INTRODUCTION 21 The Yakima fold and thrust belt (YFTB) of central Washington State is a set of 22 prominent anticlines in the Miocene Columbia River Basalt flows (CRB; figure 1). The YFTB 23 anticlines form three distinct sets (Riedel et al., 1989 and 1994; Watters, 1989). The Frenchman 24 Hills, Saddle Mountains (Riedel, 1984; West et al., 1996) and east part of Umtanum Ridge 25 (Gable Mountain) in the northeast YFTB are west-trending anticlines above south-dipping 26 reverse faults. To the south the Columbia Hills, Horse Heaven Hills, Ahtanum, Yakima and 27 Toppenish Ridges (Campbell and Bentley, 1981) form a fan-shaped pattern of southwest- to 28 west-trending ridges. In the northwest, Manastash, Umtanum and part of Yakima Ridge (Price 29 and Watkinson, 1989) trend northwest. YFTB structures are growing under N-S or NNE-SSW 30 compression (Hooper and Conrey, 1989; Reidel et al., 1994; McCaffrey et al., 2007), but their 31 variety of trends suggests that they developed in a more complex stress field. 1 32 The Olympic-Wallowa Lineament (OWL) is a northwest-trending, ~1100-km-long 33 alignment of topographic and structural features that cuts through the YFTB (figure 1; Raisz, 34 1945). In the YFTB 1 the OWL includes the Wallula fault zone (Mann and Meyer, 1993; Riedel 35 and Tolan, 1994; Mann, 1994) and the fault-bounded Manastash and Umtanum ridges. The OWL 36 has been interpreted as the northernmost strike-slip “megashear” bounding Basin and Range 37 extension (Hooper and Conrey, 1989; Mann and Meyer, 1993), but geologic evidence for 38 substantial strike-slip motion is hotly debated (Reidel and Tolan, 1994; Mann, 1994; Reidel et 39 al., 1989 and 1994; Hutter et al., 1994). The OWL itself extends across the Cascade Range 40 (Raisz, 1945; figure 1), possibly as a system of active faults (Blakely et al., 2011). 41 The YFTB clearly is related to the OWL. First, the northwest YFTB ridges form part of 42 the OWL. Second, the markedly (~25°) different YFTB trends on each side of the OWL show 43 that it influenced their formation. Third, the Columbia Hills and Horse Heaven Hills anticlines 44 terminate against the south side of the Wallula fault portion of the OWL. Formation of these two 45 anticlines required substantial (kms?) shortening south of the OWL, and the lack of comparable 46 shortening on the north side requires strike-slip motion along the Wallula fault (figure 1). 47 Their earthquake potential makes the origins of the YFTB and OWL more than just 48 academic questions. Within or near the YFTB on the Columbia and Snake Rivers are six major 49 dams. Adjacent to the OWL and within the YFTB is the Hanford nuclear site, with 56 million 50 gallons of liquid radioactive and chemical waste on site (DOE, 1996). Seismic hazard analyses 51 assume maximum earthquake magnitudes of 6.4 or less if the YFTB faults are assumed to be 52 restricted to the ~4-km-thick CRB (“thin-skinned” or “decoupled” models), but reach 53 magnitudes of 6.5 to 7.9 if the faults penetrate deep the CRB (“thick-skinned” or “coupled” 54 models) (Geomatrix Consultants, 1996). 55 Here I use computer models to show that the trends of the YFTB anticlines in relation to 56 the OWL match remarkably well the orientations of stress trajectories near the ends of vertical 57 strike-slip faults as computed using Linear Elastic Fracture Mechanics (LEFM) methods 58 (Fletcher and Pollard, 1981; Rispoli, 1981; Willemse and Pollard, 1998; Kattenhorn and 59 Marshall, 2006; Kim and Sanderson, 2006). The models suggest that the YFTB anticlines formed 60 as splay faults caused by diminishing strike-slip motion on the OWL in the Miocene, and the 61 YFTB faults remain the easiest path for slip despite the modern stress field being rotated slightly 1 (also known in the YFTB area as the Cle Elum-Wallula Lineament [CLEW]) 2 62 since the Miocene. Although some strike-slip motion on the OWL clearly continued to the 63 northwest (Blakely et al., 2011), I hypothesize that a substantial amount of slip on the OWL 64 terminated within the YFTB, where it was absorbed to form the anticlines. 65 66 67 COMPARISON OF MODEL STRESS TRAJECTORIES AND THE YFTB 68 I used a 3-dimensional boundary element code (Gomberg and Ellis, 1993) to model stress 69 trajectories at the tip of a 400-km-long, vertical strike-slip fault assuming LEFM behavior 70 (Figure 2; Pollard and Segall, 1987). The model simulates an abrupt decrease in the amount of 71 displacement along the OWL near Rattlesnake Mountain at the center of the YFTB. The fault in 72 the model was a stress-free surface divided into 80 segments to accommodate decreasing slip at 73 the fault ends. The stress-free condition assumes that faults are weak enough to relieve long-term 74 crustal strains. To determine the stress trajectories, I modeled about 9 m of dextral strike-slip 75 fault motion driven by a uniaxial remote stress oriented at 45° to the fault. This displacement 76 simulates a large earthquake and the resulting stress orientations. The model fault extended to 40 77 km depth, which is the approximate depth to Moho beneath the Columbia basin (Gao et al., 78 2011; Yang et al., 2008). The fault below seismogenic depths is assumed to be a ductile shear 79 zone. 80 The model shows that preferred fault trends distant from the main strike-slip fault are 81 nearly perpendicular (contractional) and parallel (extensional) to the regional stress direction 82 (figure 2). Near the fault tip the stress field rotates so that the maximum compressive stress is at 83 a large angle to the fault in the compressional quadrant and at a small angle in the extensional 84 quadrant. The compressional quadrant is an area of uplift and the extensional quadrant is an area 85 of subsidence. 86 There are remarkable similarities between the trends of the model stress trajectories and 87 the trends of the YFTB ridges northeast and southwest of the OWL (figure 2). I rotated the 88 model so the fault overlays the OWL, resulting in a direction of maximum compression (N9°W) 89 that is consistent with the NNW-SSE principal stress direction inferred for the early Miocene 90 YFTB from dike orientations (Hooper and Conrey, 1989). The Columbia and Horse Heaven 91 Hills south of the OWL are parallel to the model’s stress trajectories, and they terminate against 92 the OWL at angles close to the 70.5° predicted by fracture mechanics. Portions of Toppenish, 3 93 Ahtanum and Yakima Ridges likewise are parallel to the model stress trajectories. The 94 Frenchman Hills, Saddle Mountains, and Gable Mountain anticlines north of the OWL strike 95 nearly perpendicular to the model’s principal compressive stress direction. The north-trending 96 stretch of the Columbia River north of Rattlesnake Mountain is parallel to the predicted strike 97 direction for extensional fractures, suggesting that the fault inferred along the river (Reidel, 98 1984) may have normal motion. 99 The contrast in elevations on opposite sides of the OWL also is consistent with the model 100 (figure 2). Rattlesnake Mountain has some of the highest elevations in the area and an elongate 101 shape similar to that of the model’s uplift area. Southeast of Rattlesnake Mountain, uplift south 102 of the OWL between 8 and 6 Ma forced the Columbia River southeast to Wallula Gap (Reidel et 103 al. 1994), where the model predicts decreasing amounts of uplift. The Pasco Basin on the north 104 side of the OWL has been a locus of subsidence since the Miocene, and the approximate 105 dimensions of the basin are similar to that predicted by the fault termination model (figure 2; 106 Reidel et al., 1989 and 1994). 107 Umtanum and Manastash Ridges in the northwest part of the YFTB are also consistent 108 with formation near a fault tip, but they lie in the quadrant dominated by shear stresses and 109 strike-slip branch faults (figure 2; Kim and Sanderson, 2006). Both ridges form part of the OWL 110 and are bounded by reverse or oblique-slip faults that are nearly parallel to the orientation of 111 maximum shearing in the model. The Saddle Mountains and Frenchman Hills also change trend 112 to run parallel to the direction of maximum shearing west of the Columbia River.
Details
-
File Typepdf
-
Upload Time-
-
Content LanguagesEnglish
-
Upload UserAnonymous/Not logged-in
-
File Pages14 Page
-
File Size-