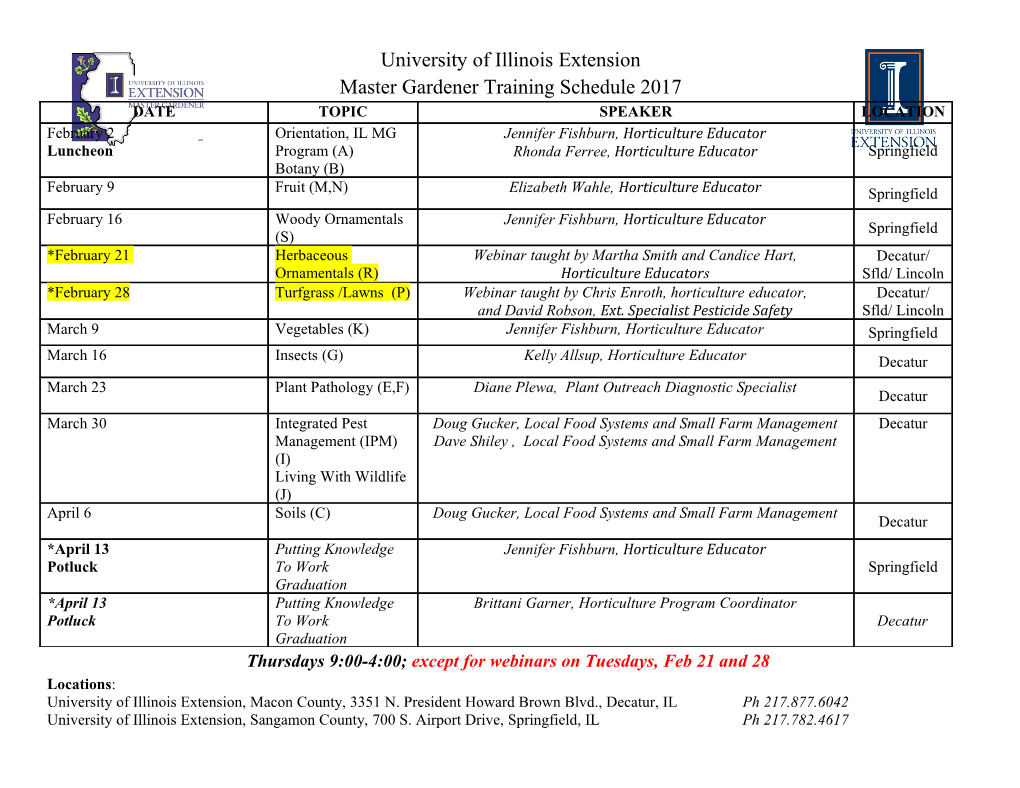
DETECTION OF THE TAIPNEIITRINO AT me LI·IC* Klaus Winter CERN. Geneva. Switzerland I. Introduction Following the discovery of the tau-lepton in e+e` collisions by M. Perl and collabo rators [1 ], all evidence today favours the hypothesis that it is a sequential heavy lepton belonging to a third lepton Family [2]. From measurements of the forward-bacl< ward angular asymmetry of tau produced in e+e` annihilations [3], [4] e+e* +* ft a value of the axial-vector neutral current coupling constant of ga = — 0.—l5 1 0.05 was deduced in agreement with ga = -1 /2 for the assignment of lel’t—handed tau leptons as the "down" state (I3 = -1 /2) of a weak isospin doublet. Hence, the most natural assignment of the "up" state (I3 = +1/2) would be to a neutrino with the same lepton flavour. the tau-neutrino. Further indirect evidence about the tau—neutrino can be obtained from leptonic tau decays [4]. e.g. 1:- Q u_v,,X. The muon energy spectrum has the familiar [2] Michel shape suggesting that the par ticle X is a spin l /2 particle. Its mass has been constrained to less than 35 MeV by analysing semi-leptonic tau—deca)s with multipion final states [5]. Hence, the particle Xmay be a neutrino. Is it a sequential neutrino, with a flavour property which distin guishes it from the electron- and the muon-neutrino? The possibility of a complete identity with we or uu can indeed be excluded. Searches for charged current neutrino reactions which produce a tau-lepton UN ·=* T_X have given negative results. A search in a beam dump experiment with vu and we at Working group on neutrino physics: L. Camilleri, A. Capone, A. De Rujula, LI. Dore, A. Eredidato, E. Fernandez, _I. Gomez, A.B. Kaidalov, P.F. Lovere, V. Palladino, F. Pietropaolo. L. Rolandi. R. Santacesaria. S. Schlenstedt, F. Vanucci and K. Winter. 37 OCR Output CERN [6] excluded the identity ve E vt by six standard deviations. A search in a muon-neutrino beam at FNAL [7] gave limits on the coupling constant of vn and t of 0.0021 and on the coupling constant oF vp and t of 0.073. Together with the life time and leptonic branching ratio measurements [3] [4], these results show that the tau—neutrin0 is distinct from ve and v Does the tau-neutrino exist as a particle? Surprisingly, this question cannot be answered by yes or no. Its existence can be proved by direct observation of the charged current reaction vTN - t" X. All attempts to directly observe the reaction have failed up to now. Searches in so—called beam dump experiments have been performed at the 450 GeV CERN—SPS. ln this type of experiment the proton beam is absorbed by a long block of copper [6]. Because of their strong absorption in copper, decays of long-lived mesons (rc and K) are suppressed by a Factor of ~l0‘. Neutrinos From decays of short—lived charm mesons (lifetime ~3‘i0`s)l3 are not suppressed. Among the known decay channels there are semiieptonic decays of the D meson (cd) into ve and vu and of the Ds meson (cs) into tvt. The contribution of the latter channel which produces tau-neu trinos is too low at the CERN proton energy of 450 GeV to be detectable. At the 800 GeV Tevatron the situation would be slightly more favourable. However, the experiments [8] have been indefinitely deferred. A new experiment [9] has been designed to detect the tau-neutrino at the 3TeV proton accelerator UNK which is presently under construction at Protvino (Serpukhov) in the LISSR. With the advent of high energy pp colliders (LHC and SSC) new possi bilities have arisen which will be discussed in the Following sections. 2. The Concept of Tau-Neutrino Detection Previous experience with the detection of short-lived particles, e.g. of charm-mesons has shown that their identificaton by kinematics and by transverse momentum unbal ance due to undetected neutrinos is not possible in the presence of a large back ground. The short lifetime itseIF is an unambiguous method to detect and to identify them. With a mean tau—neutrino event energy of 300 GeV at LHC we expect a mean decay path of ~i cm. The majority of the tau decay modes (86%) produce a single 38 OCR Output 39 charged particle. The decay angle (kink) between the tau and the charged particle is small. about 1 O on average and therefore difficult to detect. The decay length has a broad distribution because of the wide tau—neutrino energy spectrum. Because of the energy and momentum carried away in tau—decay by the vT the energy of each tau-lepton and. hence. its lifetime in the rest system cannot be determined. For the identification of a tau decay vertex we require instead a visible transverse decay length (kink) or a visible transverse impact parameter of a particle track with respect to the event vertex (figure I). Figure 2 shows the expected transverse decay length and impact parameter distribu tion with mean values of XT = 243 um and X. = 65 um. These values are nearly inva riant with respect to the spread of tau—neutrino energies. The transverse decay angle is large; a spectacular event with a t + uvnvr decay, simulated by Monte Carlo me thods is shown in figure 3 [9]. lt illustrates the basic concept. Detection of short lived particles requires good space resolution (~20 um). There exist proven methods: emulsions, holographic bubble chambers or Silicon micro-strip detectors. Other detection techniques using e.g. a liquid argon drift chamber have al so been investigated [IO]. Because of the high muon flux (see Chapter 3) it is essential to have good time resolution (E, l0 us). This condition eliminates the emulsion and the bubble chamber as detectors. The CHARM lI·—Zeuthen Collaboration has been considering either Silicon micro-strip detectors or scintillating fibres [9], others have developed liquid argon drift chambers [10] for detecting the tau- neutrino. ln Chapter 3, I am briefly describing the vt beam and the expected vt event rate in Chapter 4 the detector and in Chapter 5 the expected performance. 3. The Tau-Neutrino Beam Tau neutrinos can be obtained from the production and decay of heavy quark flavors (cc. bb) in proton-proton collisions. The main source of vT is the leptonic decay D; -» vt ++t, 1*» vt + X which is expected to occur with a branching ratio of ~3%. The secondary vt from t decay has higher energy. on average 300 GeV at LHC energy, because of the Lorentz boost of the tau lepton in DQ decay. and is there dominating the expected event rate. OCR Output At the energy of fixed target accelerators (LINK, LHC, SSC) of »/s #-80- 120GeV the cross section for DQ production can be safely estimated from results obtained at the ISR and at the pp colliders [9]. Llsing the collider mode of LHC, following a suggestion of A. De Rujula and R. Riickl [11], a ti., beam can be produced in —/s z 16 TeV pp col lisions. The cross section dependence on w/s and on the Feynman scaling variable xl: has been estimated by a scaling law approach [11], by the quark-gluon string model of Kai dalov et al. [12] and by the PYTHIA QCD Monte Carlo program. A very steeply rising xp dependence at x ~0, like 1/x and a strong cross section increase with energy is predicted. with o(D+) ~ o(Dd)~o(DS)~ 1 mb at 16 TeV, a factor ~20 higher than in the fixed target mode at 1/s ~ 120GeV. These predictions must be subjected to experimental tests. Figure #1- shows the energy spectrum of vt at —/s = 16TeV as calculated by De Rujula, Fernandez and Gomez. Because of the energy imparted to the parent DS mesons the angular distribution of the vt flux is strongly peaked at small angles with respect to the proton direction (see figure 5). The luminosity, the vt event rate for 10’s for a detector with a mass of 2 kg/cm2 subtending an angular acceptance of :2.5mrad, and the rate of v,, and vn induced reactions (from heavy quark decays) in a conventional detector with a mass of 6 kg/cm2 is given in Table 1 for three different modes of operation: a gas jet with a density of p~ 4-1014 nucleons/cm3 and a beam dump receiving 1010 protons/s by slow ejection operate in the fixed target mode; for the beam—beam mode we have assumed a mean luminosity of 103’1cm`2s`1 whereas the peak luminosity may be as high as 4-1034. For comparison we also show the rate at a fixed target accelerator (LINK) with 10protons/s.13 OCR Output Table 1 Comparison of event rates in different operation modes at the LHC and at LINK. Mode L(cm_2s`1) I N(v,)4) I N(v)S)= N(v) {i€ Beam-Beam 1034 3800 80'0O0 Gas—_]et” (fixed target) I 2·103 500 Beam dump2'(fixed iargi-;t>| 2-103 2500 50000 LINK fixed target3 1038 20'000 1) p (jet) ~ L10nucleons/cm14 2) slow ejection 10protons/s10 3) slow ejection 1013 protons/s 4) target mass ~2kg/cm2, A(E)~12.5mrad, 107s S) target mass~6kg/cm2, A@~:2.5 mrad, l07s Detecting t decays in the leptonic decay it -¢ uvHvT with a branching ratio of 17.8% and a detection efficiency (see Chapter -l-) of E~30% observation of the reaction vTN tX may be possible.
Details
-
File Typepdf
-
Upload Time-
-
Content LanguagesEnglish
-
Upload UserAnonymous/Not logged-in
-
File Pages13 Page
-
File Size-