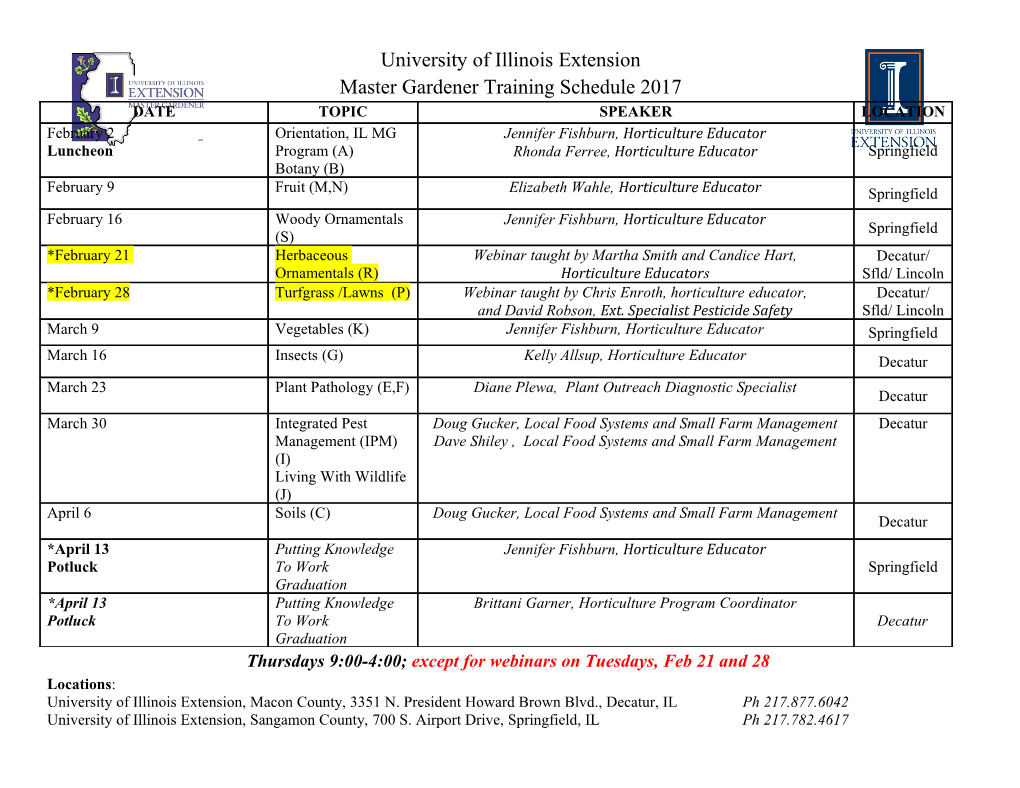
α-Actinin/titin interaction: A dynamic and mechanically stable cluster of bonds in the muscle Z-disk Marco Grisona, Ulrich Merkela, Julius Kostanb, Kristina Djinovic-Carugob,c, and Matthias Riefa,d,1 aPhysik Department E22, Technische Universität München, 85748 Garching, Germany; bDepartment of Structural and Computational Biology, Max F. Perutz Laboratories, University of Vienna, A-1030 Vienna, Austria; cDepartment of Biochemistry, Faculty of Chemistry and Chemical Technology, University of Ljubljana, SI-1000 Ljubljana, Slovenia; and dMunich Center for Integrated Protein Science, 81377 Munich, Germany Edited by James A. Spudich, Stanford University School of Medicine, Stanford, CA, and approved December 16, 2016 (received for review August 2, 2016) Stable anchoring of titin within the muscle Z-disk is essential for In humans, the isoforms of titin exhibit four to seven Z-repeats preserving muscle integrity during passive stretching. One of the (15, 16, 20). The structure of the EF3-4 hands complex with titin main candidates for anchoring titin in the Z-disk is the actin cross- Z-repeat 7 shows the bound Z-repeat in an α-helical confor- linker α-actinin. The calmodulin-like domain of α-actinin binds to mation (21). In solution assays, binding affinities of various the Z-repeats of titin. However, the mechanical and kinetic prop- Z-repeats to EF3-4 were determined to lie in the micromolar erties of this important interaction are still unknown. Here, we use range (22). Micromolar affinity points only to a moderately a dual-beam optical tweezers assay to study the mechanics of this stable interaction, the kinetics of which are unknown. This raises interaction at the single-molecule level. A single interaction of the question of how such a relatively weak interaction can α -actinin and titin turns out to be surprisingly weak if force is achieve the task of firmly anchoring titin within the Z-disk even applied. Depending on the direction of force application, the un- under applied mechanical loads. To answer this question, we set binding forces can more than triple. Our results suggest a model out to investigate the mechanical as well as kinetic stability of α where multiple -actinin/Z-repeat interactions cooperate to en- this interaction directly in a single-molecule mechanical experi- sure long-term stable titin anchoring while allowing the individual ment. We provide evidence that the concerted action of several components to exchange dynamically. α-actinin/Z-repeat bonds can establish long-term mechanically stable anchoring, whereas the individual bonds can break and α-actinin | titin Z-repeats | Z-disk mechanics | optical tweezers reform on the second timescale. uscle is the tissue that is constantly subjected to high me- Results Mchanical loads. Whereas thick and thin filaments are re- Interaction Between α-Actinin and Titin Z-Repeat 7 (PullA-T7 Geometry). sponsible for active force production, the passive elasticity of To probe the mechanical strength of the interaction between muscle is dominated by titin/connectin filaments (1). Hence, α-actinin and titin Z-repeat 7 (T7), we prepared a construct where under passive stretching conditions the integrity of muscle relies 23 residues of T7 (21) were fused to the C terminus of EF3-4 of the ’ on titin s being firmly anchored within the sarcomere, preventing CaMD of α-actinin 2 via a 4 × (GGS) linker. To apply load to this A the interdigitated muscle filaments from falling apart (Fig. 1 ). fusion construct we tethered dsDNA linkers to cysteine residues at Whereas titin is firmly attached to thick filaments in the A-band the termini (Fig. 1C) that could be attached to 1-μm-sized beads in – and the M-line (2 6), it is much less clear how stable anchoring is the optical tweezers. For details on the assay see Materials and achieved in the Z-disk, where adjacent sarcomeres overlap. The Methods and SI Materials and Methods. Note that in this construct superstable titin/telethonin interaction within the Z-disk was α – the force propagates through both the -actinin EF3-4 domain and considered important for titin anchoring (7 9), but knockout the T7 peptide, mimicking how force propagates in the Z-disk (Fig. mutants later showed that it is not essential for muscle integrity 1B). We therefore called this construct PullA-T7. (10–12). Apart from a direct interaction between actin filaments BIOPHYSICS AND and titin at the Z-disk edge (13), the most prominent candidate COMPUTATIONAL BIOLOGY Significance for the anchoring of titin within the Z-disk is its interaction with α-actinin (Fig. 1B) (6, 12, 14). Four isoforms of human α-actinin have been identified: the Muscle is the tissue in our body experiencing most extreme calcium-insensitive muscle isoforms 2 and 3, which cross-link mechanical forces. The mechanism of active force generation actin filaments in sarcomere-delimiting Z-disk complexes, and has been investigated for more than 50 y and is fairly well calcium-sensitive nonmuscle isoforms 1 and 4. α-Actinin is an understood. However, despite its physiological significance, it antiparallel homodimer whose most prominent task is cross- is still unknown what mechanical linkages hold together the linking actin filaments of neighboring sarcomeres in the Z-disk muscle machinery under passive stretching forces. In this pa- B per, we show with direct mechanical single-molecule mea- (Fig. 1 ; reviewed in ref. 14). In each subunit, a flexible region α called the neck separates the actin binding domain (ABD) from surements that an array of titin/ -actinin bonds composes a four spectrin-like repeats (SR) forming the rod region (Fig. 1B dynamic network that can provide stable anchoring, main- and Fig. S1). The rod regions of the two subunits interact and taining the integrity of the muscle Z-disk even under load. This dynamic network explains how components of the Z-disk are provide a rigid spacer between the actin filaments. At the other able to rapidly rearrange and, at the same time, form a long- end of each subunit a calmodulin-like domain (CaMD) formed term stable mechanical structure. by two pairs of EF-hands (EF1-2 and EF3-4) is able to bind a – Z-disk region of titin formed by the so-called Z-repeats (15 17). Author contributions: M.G., K.D.-C., and M.R. designed research; M.G. performed re- The current model for α-actinin 2 dynamic regulation suggests search; U.M. and J.K. contributed new reagents/analytic tools; M.G. and M.R. analyzed that EF3-4 hands of one subunit bind to the neck region of the data; and M.G., K.D.-C., and M.R. wrote the paper. juxtaposed subunit, thus not being available for the interaction The authors declare no conflict of interest. with titin Z-repeats (Fig. S1) (18, 19). Upon activation of α-actinin This article is a PNAS Direct Submission. in the Z-disk by phosphatidylinositol 4,5-biphosphate (PIP2), EF3-4 1To whom correspondence should be addressed. Email: [email protected]. is released from the neck and binding of titin can be achieved in this This article contains supporting information online at www.pnas.org/lookup/suppl/doi:10. open conformation of α-actinin. 1073/pnas.1612681114/-/DCSupplemental. www.pnas.org/cgi/doi/10.1073/pnas.1612681114 PNAS | January 31, 2017 | vol. 114 | no. 5 | 1015–1020 Downloaded by guest on September 26, 2021 A D B E F G H I C Fig. 1. Interaction between α-actinin EF3-4 and titin Z-repeat 7 in PullA-T7 geometry. (A) Schematic of the sarcomere organization under stretching con- ditions, showing titin elongation. (B) Arrangement of actin, titin, telethonin, and α-actinin within the Z-disk. (C) Schematic of the dual-beam optical tweezers experimental setup with a fusion construct [Protein Data Bank (PDB) ID code 1h8h]. Yellow dots mark cysteine residues, here and elsewhere. (D) Force- extension trace obtained by moving the beads apart at a constant velocity, here for the PullA-T7 fusion construct. Gray dots are full bandwidth data, and black dots are smoothed data. Dotted lines are WLC fits (SI Materials and Methods). (E) Passive-mode time trace of 5 s at an average force of 3.7 pN. Gray dots are full-bandwidth data, on which HMM analysis was performed assuming the kinetic network on the right. For clarity, smoothed data are colored, based on HMM-assigned states. (Top Right) Zoom of 1 s, where full-bandwidth data are colored and smoothed data are in black. (F) Force-dependent transition rates from UU to FU (red) and from FU to UU (green). Solid lines are fits that allow extrapolation to zero-force rates (SI Materials and Methods). (G) Same for transition rates from FB to FU (purple) and from FU to FB (green). (H) Population probability of the three states as a function of force, and fits as described in SI Materials and Methods.(I) Competition assay performed by addition of T7 in solution to evaluate the dissociation constant. A passive-mode sample trace of 5 s shows that the solution binding event (cyan) has the same force (and contour length) as the FU state but can be identified by the different lifetime. When force is applied to PullA-T7, unbinding/unfolding We used a hidden Markov model (HMM) algorithm (23) to transitions occur already at surprisingly low forces, as shown in identify the transitions between this three-state system on the Fig. 1D. At the low pulling speed of 10 nm/s, only transitions unfiltered traces. From the dwell-time distributions we calculated between the fully folded/peptide-bound state and the unfolded/ transition rates assuming the transition network shown in Fig.
Details
-
File Typepdf
-
Upload Time-
-
Content LanguagesEnglish
-
Upload UserAnonymous/Not logged-in
-
File Pages6 Page
-
File Size-