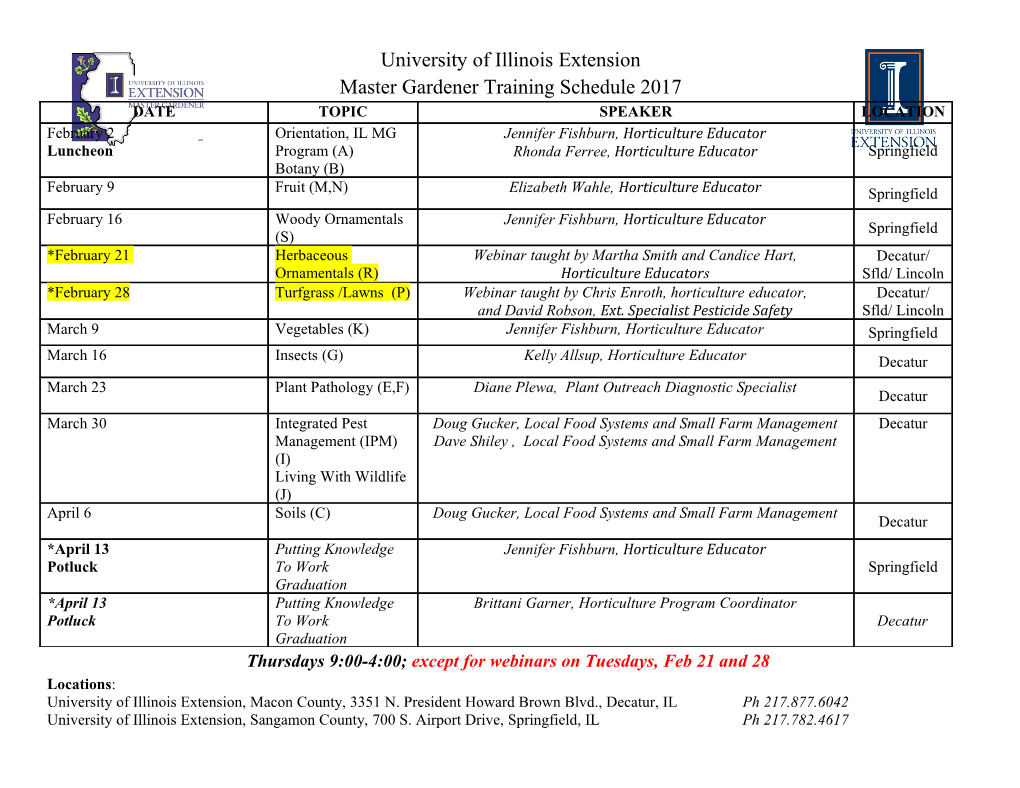
Aspects of Advanced Fuel FRC Fusion Reactors John F Santarius and Gerald L Kulcinski Fusion Technology Institute Engineering Physics Department CT2016 Irvine, California August 22-24, 2016 [email protected]; 608-692-4128 Laundry List of Fusion Reactor Development Issues • Plasma physics of fusion fuel cycles • Engineering issues unique or more ! Cross sections and Maxwellian reactivity important for DT fuel ! Beta and B-field utilization ! Tritium-breeding blanket design ! Plasma fusion power density ! Neutron damage to materials ! Plasma energy and particle confinement ! Radiological hazard (afterheat and waste disposal) ! Neutron production vs Ti for various fuel ion ratios • Safety • Geometry implications for engineering design • Environment ! Power flows • Licensing ! Direct energy conversion ! Magnet configuration • Economics ! Radiation shielding ! Maintenance in a highly radioactive environment • Nuclear non-proliferation ! Coolant piping accessibility • Non‑electric applications • Plasma‑surface interactions • 3He fuel supply JFS 2016 Fusion Technology Institute, University of Wisconsin 2 UW Developed and/or Participated in 40 MFE & 26 IFE Power Plant and Test Facility Studies in Past 46 years MFE-40 IFE-26 JFS 2016 Fusion Technology Institute, University of Wisconsin 3 Total Fusion Reactivities for Key Fusion Fuels Total Energy Production Rate st 1 generation fuels: MaxwellianTotal Reactivities -19 D + T → n (14.07 MeV) + 4He (3.52 MeV) 10 D + D → n (2.45 MeV) + 3He (0.82 MeV) → p (3.02 MeV) + T (1.01 MeV) -20 {50% each channel} 10 D-T D-3He -21 2nd generation fuel: 10 D + 3He → p (14.68 MeV) + 4He (3.67 MeV) 10-22 rd 3 generation fuels: p-11B 3He + 3He 2 p + 4He (12.86 MeV) → 10-23 3He- 11 4 - 3 p + B → 3 He (8.68 MeV) D D He 10-24 100 101 102 Ion temperature keV JFS 2016 Fusion Technology Institute, University of Wisconsin 4 H L What Are the Solar System 3He Resources? • ~100 kg 3He accessible on Earth ! ~2 GW-y fusion energy for R&D • ~109 kg 3He on lunar surface for the 21st & 22nd centuries ! ~1000 y world energy supply • ~1023 kg 3He in gas-giant planets for the indefinite future ! ~1017 y of world energy supply L.J. Wittenberg, J.F. Santarius, and G.L. Kulcinski, “Lunar Source of 3He for Commercial Fusion Power,” FusionEscher, Technology Other 10, 167 (1986).World, 1947 JFS 2016 Fusion Technology Institute, University of Wisconsin 5 Private Enterprise Has Discovered the Moon Lunar Missions 2003-2021 JFS 2016 Fusion Technology Institute, University of Wisconsin 6 At a Minimum, the Fusion Power Must Sustain the Plasma against Charged-Particle and Bremsstrahlung Losses • Ignition against bremsstrahlung (only) is For Te = Ti calculated using 1024 ! Bremsstrahlung formulas " Nonrelativisticq ne 3 ni nk 3 P = Pbrem#43! eff 2 T0.5e + Ti fus! " ΤeE 2 k nΤ 2 Pbrem 5.36 10 Z ne Te kE 1023 D!D " Relativistic P ! 5.36 " 10#43 n2 T0.5 0.00414 T $ 0.071 Zeff T#0.5 3 brem e e e 3 e " 22 He:D " # s $ eff $ $ " #6 2 3 10 Z 1 0.00155 Te 7.15!10 Te ! "1:1 m ! " Definitions ! E Τ 21 z2 n z3 n e 10 eff i" i eff i i #$ n Z % and Z3 % ne ne i i D!T 1020 % % • Note: fusion ash accumulation, an issue for all reactor plasmas, possibly will be 1019 1 5 10 50 100 mitigated in FRCs due to nonadiabicity of Ti keV the several MeV energy of the fusion- 3 product alphas (and protons for D He). ! " JFS 2016 Fusion Technology Institute, University of Wisconsin 7 Neutron Production & Plasma Fusion Power Density Neutron Production Relative Power Density at Constant B & β Maxwellian Plasma 1. 0.5 D!T 0.2 0.1 0.05 0.02 3He:D"1:1 Watershed level for no 0.01 blanket/shield changeout 0.005 D!D Relative fusion power density T burn fraction = 0.5 0.002 for D-D & D-3He 0.001 1. 2. 3. 5. 7. 10. 20. 30. 50. 70.100. Ion temperature keV Pplasma 2nkBT ! " 2 β ≡ ≈ 2 ⇒ n ∝ βB PBfield B /2µ0 P fus = n n E < σ v > ∝ β2 B4 V 1 2 fus € JFS 2016 Fusion Technology Institute, University of Wisconsin 8 € 3He-3He Reactions Will Produce Less Radioactivity than p-11B Reactions in Maxwellian Plasmas JFS 2016 Fusion Technology Institute, University of Wisconsin 9 Advanced Fuel Fusion Power Density Can Improve Greatly through Increasing β and B-Field • Low tokamak and stellarator β limits cause optimized reactor design B-fields to approach the technological limits (~20 T) on magnet coils, leaving little room to gain power density by increasing B. • High-β concepts optimize at low B, because neutron damage requires frequent blanket and shield changeout, leaving a large technical margin for increasing B. JFS 2016 Fusion Technology Institute, University of Wisconsin 10 Advancd-Fuel Full-Lifetime Structure Gives a More Robust System and Reduces Maintenance Frequency JFS 2016 Fusion Technology Institute, University of Wisconsin 11 Linear Geometry Allows Much Easier Maintenance and Plumbing • Some advantages of linear ITER Fusion Core geometry: ! Few interlocking systems ! Modularity ! Easier routing of plumbing Tri Alpha Energy C-2 Experiment JFS 2016 Fusion Technology Institute, University of Wisconsin 12 Power Flows Can Be Handled Much More Easily in Linear Geometry • Charged-particle power transports from internal plasmoid (in an FRC or spheromak) to edge region and then out ends of fusion core. • Expanded flux tube in end chamber reduces heat and particle fluxes. • Mainly bremsstrahlung power contributes to first-wall surface heat. • Relatively small peaking factor along axis for bremsstrahlung and neutrons. JFS 2016 Fusion Technology Institute, University of Wisconsin 13 Direct Energy Conversion Can Be Applied to End-Loss Plasma Barr-Moir experiment, LLNL • Experiment and theory agreed (Fusion Technology, 1973) within 2%. • Direct conversion would provide 60-80% efficiency for escaping fusion products. Ion beam • Bremsstrahlung (x-rays) require thermal energy conversion. JFS 2016 Fusion Technology Institute, University of Wisconsin 14 Plasma Surface Interactions Can Create Damage + • Linear geometry devices can 30 keV He Irradiation of Polycrystalline Tungsten handle high heat fluxes, caused 17 + by charged particles escaping φL - 6x10 He / cm2 at 900 oC. the core, by expanding the flux tube in the end tank. • Tokamaks take that heat and those particles mainly in a thin strip along the divertor, leading to surface heat fluxes of 10-20 MW m-2. 3 µm 200 nm From S. J. Zenobia, L. M. Garrison, G. L. Kulcinski. "The response of polycrystalline tungsten to 30 keV helium ion implantation at normal incidence and high temperatures." Journal of Nuclear Materials 425, 83 (2012). JFS 2016 Fusion Technology Institute, University of Wisconsin 15 Can We Design Proliferation-Proof Advanced Fuel FRCs? Large α particle & D3He Direct converter for Advanced fuel for proton gyroradii contribute increased electric low neutron wall loading to macroscopic stability power per unit High β for high fusion fusion power power density Minimal Superconducting, radiation shield high-field magnet Organic coolant to to reduce for high fusion make high-flux DT space for DT power density operation difficult shielding JFS 2016 Fusion Technology Institute, University of Wisconsin 16 Summary • DT fuel is the easiest to burn, whereas burning advanced fuels requires continued, modest plasma physics progress, especially in energy confinement. • Physics development path typically costs less than engineering development path => advanced fuels. • Considerations of engineering, safety, environment, and licensing favor advanced fuels, while cost remains to be determined. • Advanced fuels require the development of the FRC or another suitable high‑β, innovative concept. JFS 2016 Fusion Technology Institute, University of Wisconsin 17 Back Pocket Slides 3He Plays a Key Role in the Advanced Fusion Fuels -27 Key Fusion Fuels 10 1st Generation D-T 10-28 D + T → n (14.07 MeV) + 4He (3.52 MeV) 3 D + D → n (2.45 MeV) + 3He (0.82 MeV) D- He 10-29 → p (3.02 MeV) + T (1.01 MeV) Total {50% each channel} D-D p-11B 10-30 2nd Generation D + 3He p (14.68 MeV) + 4He (3.67 MeV) → 10-31 3He-3He 3rd Generation 10-32 p + 11B → 3 4He (8.68 MeV) 1. 2. 5. 10. 20. 50. 100. 200. 500.1000. Center-of-Mass Energy keV 3He + 3He → 2 p + 4He (12.86 MeV) H L JFS 2016 Fusion Technology Institute, University of Wisconsin 19 Advanced Fuels Could Lower R&D Costs Engineering R&D costs typically dominate physics R&D costs. JFS 2016 Fusion Technology Institute, University of Wisconsin 20 .
Details
-
File Typepdf
-
Upload Time-
-
Content LanguagesEnglish
-
Upload UserAnonymous/Not logged-in
-
File Pages20 Page
-
File Size-