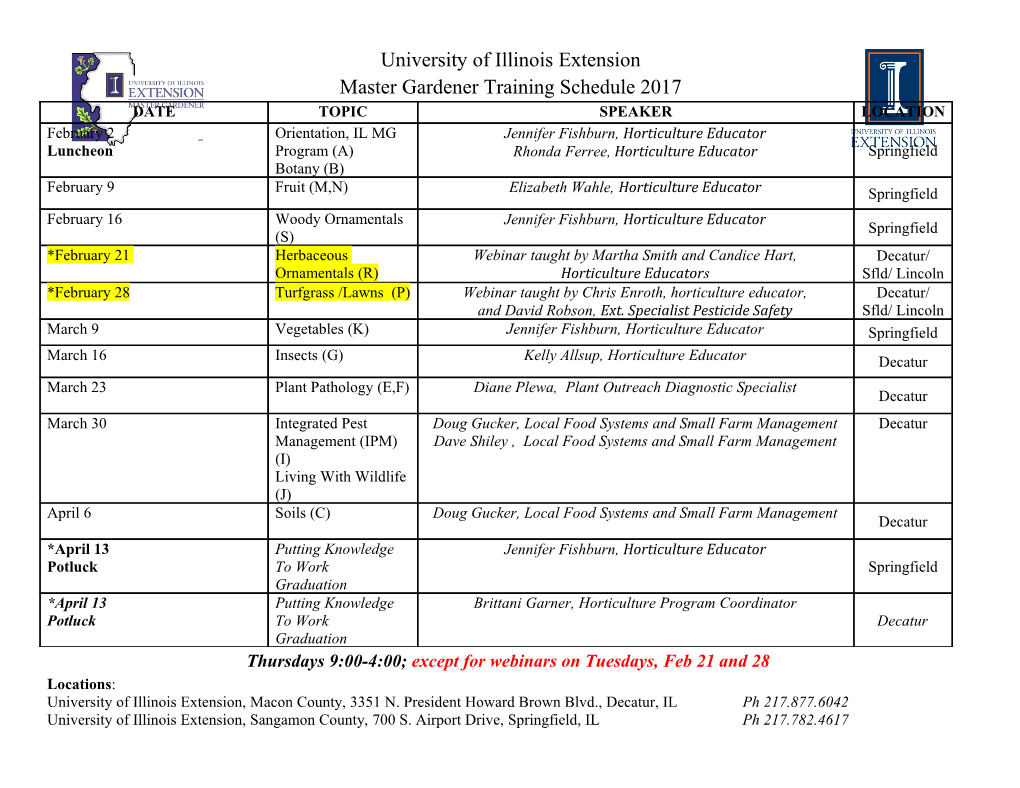
FACULTY OF VETERINARY MEDICINE approved by EAEVE The Role of Joint Biomechanics in the Development of Tarsocrural Osteochondrosis in Dogs WALTER BENJAMIN DINGEMANSE Thesis submitted in fulfilment of the requirements for the degree of Doctor of Philosophy (PhD) in Veterinary Sciences, Faculty of Veterinary Medicine, Ghent University 2017 Promoters: Dr. Ingrid Gielen Prof. Dr. Ilse Jonkers Prof. Dr. Bernadette Van Ryssen Prof. Dr. Magdalena Müller-Gerbl Department of Veterinary Medical Imaging and Small Animal Orthopaedics Faculty of Veterinary Medicine Ghent University The Role of Joint Biomechanics in the Development of Osteochondrosis in Dogs Walter Benjamin Dingemanse Vakgroep Medische Beeldvorming van de Huisdieren en Orthopedie van de Kleine Huisdieren Faculteit Diergeneeskunde Universiteit Gent Cover design by Michael Edmond Nico Van Waegevelde © Walter Benjamin Dingemanse 2017. No part of this work may be reproduced or transmitted in any form or by any means without permission from the author. PhD supported by a grant from IWT I MAY NOT HAVE GONE WHERE I INTENDED TO GO BUT I THINK I HAVE ENDED UP WHERE I NEEDED TO BE - DOUGLAS ADAMS - EXAMINATION BOARD Chair: Prof. dr. Hilde de Rooster Faculty of Veterinary Medicine, Ghent University, BE Secretary: Prof. dr. Wim Van den Broeck Faculty of Veterinary Medicine, Ghent University, BE Members: Prof. dr. Robert Colborne Institute of Veterinary, Animal & Biomedical Sciences, Massey University, NZ Dr. Evelien de Bakker Faculty of Veterinary Medicine, Ghent University, BE Dr. Maarten Oosterlinck Faculty of Veterinary Medicine, Ghent University, BE Prof. dr. Benedicte Vanwanseele Faculty of Kinesiology and Rehabilitation Sciences, KU Leuven, BE Prof. dr. Jos Vander Sloten Faculty of Engineering Science, KU Leuven, BE I TABLE OF CONTENTS EXAMINATION BOARD I TABLE OF CONTENTS III LIST OF ABBREVIATIONS VIII GENERAL INTRODUCTION CHAPTER ONE 3 SUBCHONDRAL BONE – MORPHOLOGY, DENSITY, AND DENSITY EVALUATION CHAPTER TWO 15 DIAGNOSIS AND TREATMENT OF TARSOCRURAL OSTEOCHONDROSIS IN DOGS CHAPTER THREE 43 COMPARISON OF MORPHOLOGICAL AND CLINICAL FEATURES BETWEEN MEDIAL AND LATERAL TROCHLEAR RIDGE TALAR OSTEOCHONDRITIS DISSECANS IN DOGS CHAPTER FOUR 63 SCIENTIFIC AIMS III CHAPTER FIVE 69 SUBCHONDRAL BONE DENSITY DISTRIBUTION OF THE TALUS IN CLINICALLY NORMAL LABRADOR RETRIEVERS CHAPTER SIX 89 DENSITY AND STRENGTH DISTRIBUTION OF THE SUBCHONDRAL BONE PLATE OF THE CANINE TALUS CHAPTER SEVEN 107 A PROSPECTIVE FOLLOW UP OF AGE RELATED CHANGES IN THE SUBCHONDRAL BONE DENSITY OF THE TALUS OF HEALTHY LABRADOR RETRIEVERS CHAPTER EIGHT 123 SUBCHONDRAL BONE DENSITY CHANGES OF THE TALUS IN DOGS WITH TARSOCRURAL OSTEOCHONDROSIS CHAPTER NINE 143 SPATIAL SUBCHONDRAL BONE DENSITY REFLECTING JOINT LOADING OF THE TALUS IN DIFFERENT CANIDAE CHAPTER TEN 161 QUANTIFICATION OF JOINT LOADING IN THE HIND LIMBS OF THE LABRADOR RETRIEVER (CANIS FAMILIARIS) USING A THREE-DIMENSIONAL MUSCULOSKELETAL MODEL IV CHAPTER ELEVEN 191 FUTURE PERSPECTIVES: EVALUATION OF JOINT LOADING DISTRIBUTION IN THE TARSOCRURAL JOINT BY MEANS OF FINITE ELEMENT MODELING CHAPTER TWELVE 205 GENERAL DISCUSSION SUMMARY 231 SAMENVATTING 239 BIBLIOGRAPHY 249 DANKWOORD 259 V VI LIST OF ABBREVIATIONS 3D three-dimensional ACLT anterior cruciate ligament transsection CT computed tomography DOF degrees of freedom FOV field of view GRF ground reaction force HU Hounsfield Unit ID inverse dynamics IK inverse kinematics kV kilovoltage L lateral LCS local coordinate system mAs milliampere-seconds MPR multi-planar reconstruction MRI magnetic resonance imaging MSM musculoskeletal model M medial N Newton N/A not available OC osteochondrosis PMMA polymethylmethacrylate PSCA physical cross sectional area ROI region of interest SO static optimization VII GENERAL INTRODUCTION CHAPTER ONE Subchondral Bone – Morphology, Density, and Density Evaluation W. Dingemanse 1, M. Müller-Gerbl 2, H. van Bree 1, I. Gielen 1 1 Department of Medical Imaging of Domestic Animals and Orthopaedics of Small Animals Faculty of Veterinary Medicine, Ghent University. Merelbeke, Belgium 2 Institute of Anatomy, Basel University. Basel, Switzerland CHAPTER 1 BONE AND BONE ADAPTATION Bones provide a solid structure for muscle attachment and protect the most vital organs. Bones are also made of living cells that function like an organ, whose structure continually changes, and adapts to current needs. Mature bone tissue is composed of 60–70% mineral substance and 30–40% organic matrix, mainly (85– 90%) type I collagen fibrils. The osteoblasts are the main cells responsible for bone formation and secrete important constituents of the extra-cellular matrix (ECM). Calcium hydroxyl apatite crystals are deposited within the triple helix of the collagen type I fibrils 1. The mineral substance does not only have a biomechanical role (strength), it also has an important metabolic role (mineral homeostasis and reservoir function for many different ions) 2. The mechanical loads and associated strains, help to control and guide bone morphology 3. This response to bone strains not only dictates general bone anatomy, it also allows for adaptations to changes in loading. The adaptations are done through modeling and remodeling of bone. This is a result of local strains either staying below the remodelling threshold or exceeding the modelling threshold 3. The loading of bones leads to a certain degree of deformation, even though this degree is low, since it is a hard, mineralised tissue. The deformation generates strains, and when these strains exceed the modeling threshold, bone modeling is signalled to increase bone strength (locally), and thereby reducing later strains 3. The need for these adaptations is signalled through the different bone cells, which are mechanosensitive 4. The conversion of a mechanical stimulus to into a cellular response is called mechanotransduction 5. The four important cells in bone include (1) osteoclasts, which are of hematopoietic origin and resorb bone, (2) osteoblasts, mesenchymal-derived and form bone, (3) terminally differentiated osteoblasts embedded in the bone, called osteocytes, and (4) the mesenchymal stem cell (MSC), which are the osteoprogenitor cells 4. These cells can detect mechanical signals independently and interact with each other through different pathways to regulate bone modeling and remodeling. The osteocytes form a network through their 5 CHAPTER 1 canalicular projections, and the movement of fluid within these canaliculi generates shear forces that play a role in the mechanotransduction of bone loading to cell-level information cells 4. On a cell level, the surface proteoglycan layer is the primary sensor of mechanical signals. The cell membrane deformations are transmitted to the cytoskeleton through integrin and cadherin proteins 5. The different intra-cellular signalling pathways that are part of the adaptive response are beyond the scope of this work, they have however been described in detail elsewhere 5-7. SUBCHONDRAL BONE Definitions The subchondral bone plate is defined as the bony lamella immediately below the calcified articular cartilage. The line separating these two structures is known as the ‘cement line’. At the level of the cartilage, a second line i.e. the ‘tidemark’ separates the articular cartilage and the underlying calcified articular cartilage 8. The subchondral bone plate receives additional support from the subchondral trabeculae and which are grouped together as ‘subchondral bone’ 8. Morphology The subchondral bone plays an important role in the transfer of loads across the joint surface. Thus, the morphology of the subchondral bone is dictated by the compressive forces acting upon it. As in all bones, the structure is continually adapted to current needs. At the level of the joints, these needs are predominantly the transduction of (compressive) forces from the proximal to the distal bones forming a specific joint 9,8. These forces give rise to local strains and stresses and induce modelling and remodelling of the subchondral bone, as discussed above. The thickness of the subchondral bone plate varies among joint and has regional variations within a joint 8. These variations in thickness are associated with joint loading and the loading regime 10 and have been correlated with joint pathology 11. 6 CHAPTER 1 Density The density of the subchondral bone at any given time is the result of the bone functional adaptation 8,12. It is a heterogeneously mineralized material described by the apparent density and the mineral density. ����������� ���� �������� ������� = ������ ������ ����������� ���� �������� ������� = ������ �� �ℎ� �������� ������ The difference between these two density measures is geometrical, and is due to the presence of pores 13,14. The biomechanical properties of the subchondral bone are characterized by mineral density, collagen content, and ash fraction 14. In general subchondral bone density can be adapted by changing the apparent density, the material density, or a combination of both. These biomechanical properties can change through modeling and remodeling of the articulating bones, guided by local strains 7,15. The material properties are tissue level properties and by definition independent of the size of the bone 14. Increased joint loading leads to increased local strains and bone modelling ensures an increase in subchondral bone density to withstand the increased loading 3,16. In addition, altered joint biomechanics lead to altered joint loading distribution, leading in turn to alterations in the
Details
-
File Typepdf
-
Upload Time-
-
Content LanguagesEnglish
-
Upload UserAnonymous/Not logged-in
-
File Pages290 Page
-
File Size-