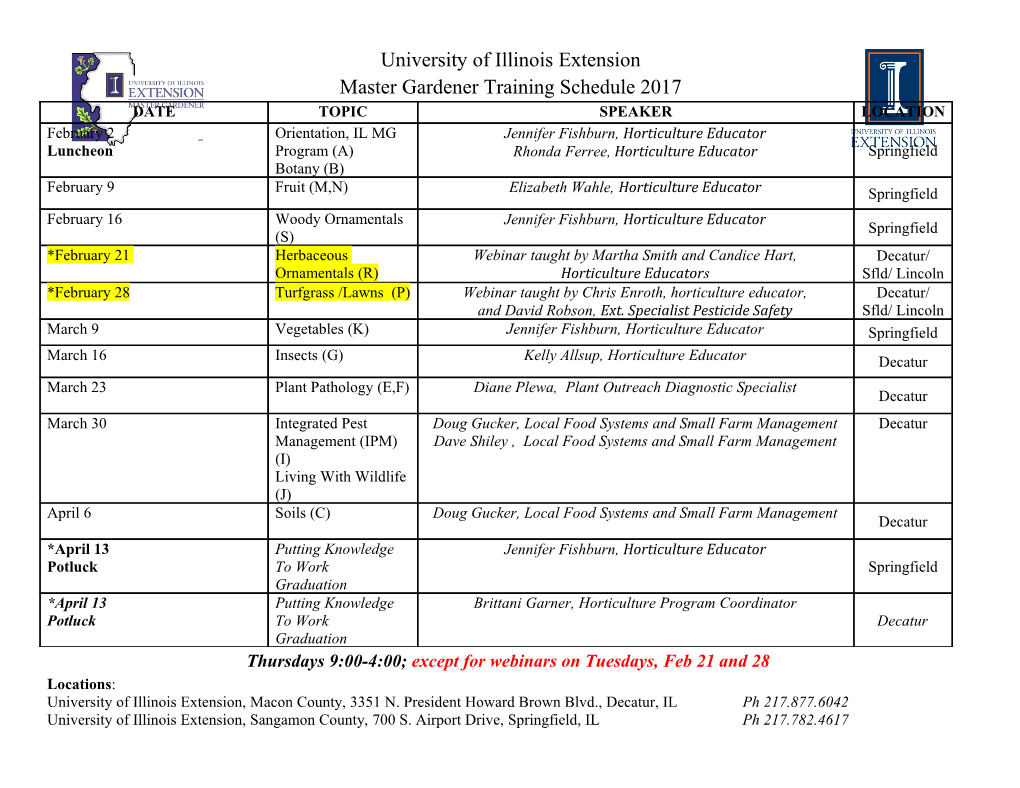
PM10CH08-Ingber ARI 2 December 2014 10:14 Engineered In Vitro Disease Models Kambez H. Benam,1 Stephanie Dauth,1,2 Bryan Hassell,1,2 Anna Herland,1 Abhishek Jain,1 Kyung-Jin Jang,1 Katia Karalis,1,3,4 Hyun Jung Kim,1 Luke MacQueen,1,2 Roza Mahmoodian,1,2 Samira Musah,1 Yu-suke Torisawa,1 Andries D. van der Meer,1 Remi Villenave,1 Moran Yadid,1,2 Kevin K. Parker,1,2 and Donald E. Ingber1,2,5 1Wyss Institute for Biologically Inspired Engineering at Harvard University, Boston, Massachusetts 02115; email: [email protected] 2Harvard School of Engineering and Applied Sciences, Cambridge, Massachusetts 02139 3Division of Endocrinology, Boston Children’s Hospital, Boston, Massachusetts 02115 4Center for Clinical, Experimental Surgery and Translational Research, Biomedical Research Foundation Academy of Athens (BRFAA), 11527 Athens, Greece 5Vascular Biology Program and Departments of Pathology and Surgery, Boston Children’s Hospital and Harvard Medical School, Boston, Massachusetts 02115 Annu. Rev. Pathol. Mech. Dis. 2015. 10:195–262 Keywords The Annual Review of Pathology: Mechanisms of disease model, tissue engineering, 3D culture, organ-on-a-chip, Disease is online at pathol.annualreviews.org microfluidic, in vitro tool This article’s doi: 10.1146/annurev-pathol-012414-040418 Abstract Copyright c 2015 by Annual Reviews. The ultimate goal of most biomedical research is to gain greater insight into All rights reserved mechanisms of human disease or to develop new and improved therapies or diagnostics. Although great advances have been made in terms of developing disease models in animals, such as transgenic mice, many of these models fail to faithfully recapitulate the human condition. In addition, it is difficult to identify critical cellular and molecular contributors to disease or to vary them independently in whole-animal models. This challenge has attracted Access provided by Harvard University on 08/10/15. For personal use only. the interest of engineers, who have begun to collaborate with biologists to leverage recent advances in tissue engineering and microfabrication to de- Annu. Rev. Pathol. Mech. Dis. 2015.10:195-262. Downloaded from www.annualreviews.org velop novel in vitro models of disease. As these models are synthetic systems, specific molecular factors and individual cell types, including parenchymal cells, vascular cells, and immune cells, can be varied independently while si- multaneously measuring system-level responses in real time. In this article, we provide some examples of these efforts, including engineered models of diseases of the heart, lung, intestine, liver, kidney, cartilage, skin and vas- cular, endocrine, musculoskeletal, and nervous systems, as well as models of infectious diseases and cancer. We also describe how engineered in vitro models can be combined with human inducible pluripotent stem cells to enable new insights into a broad variety of disease mechanisms, as well as provide a test bed for screening new therapies. 195 PM10CH08-Ingber ARI 2 December 2014 10:14 INTRODUCTION The ultimate goal of virtually all biomedical research is to understand the molecular basis of human disease in order to develop new and more effective modes of diagnosis, prevention, or therapeutic intervention. Because it is not possible to carry out fundamental research investigations using humans as “guinea pigs,” scientists have had to develop alternative models of human disease. Most commonly, animal models, such as transgenic mice with specific gene alterations, are used for this purpose. Although many of these animal models reconstitute disease manifestations and phenotypes that are ostensibly similar to those observed in humans, recent studies in sepsis, for example, show that the underlying molecular mechanisms can actually differ greatly between mice and humans with the same disease phenotype (1). This inability to effectively mimic human disease is becoming evident in increasing numbers of animal models, and it is likely the reason why many drugs fail to show efficacy and safety when advanced from animal studies to human clinical trials. For this reason, many investigators and pharmaceutical companies have placed greater emphasis on studies with cultured human cells, including primary cells, established cell lines, and, more recently, derivatives of induced pluripotent stem cells (iPSCs). Some cells cultured on standard culture dishes might exhibit differentiated cell functions, but they commonly fail to mimic tissue- and organ-level structures and functions that are central to disease etiology. Thus, there is a great need to develop alternative experimental systems containing living human cells that recapitulate tissue- and organ-level pathophysiology in vitro. In this article, we review recent advances in the development of in vitro disease models made by leveraging recent advances in tissue engineering and microfabrication. Human diseases are hugely complex, and different responses can be observed in the same organ depending on disease location, influences of the physical and chemical microenvironment, immune and inflammatory responses, and whether the condition is acute or chronic; disease manifestations can also differ depending on genetic variation between different patients. For these reasons, it will be difficult to model every facet of human disease in vitro. However, the goal in this emerging field of engineered disease models is not to solve all these problems at once. Rather, investigators take a synthetic approach by starting to build simple tissue and organ models and then constructing progressively more complex in vitro experimental systems that recapitulate more and more features that are critical for disease etiology and progression. Importantly, although this field is still in its infancy, there already have been a few exciting examples of major successes in terms of both mimicking organ-level functions and recapitulating key features of human disease in vitro. Below, we describe multiple examples of engineered in vitro disease models ranging from two-dimensional (2D) networks composed of neuronal cells derived from human iPSCs to three-dimensional (3D) reconstructions Access provided by Harvard University on 08/10/15. For personal use only. of complex heart valve structures lined by living valvular endothelial cells. In addition, we describe recently developed organ-on-a-chip cell culture devices, created with microchip manufacturing Annu. Rev. Pathol. Mech. Dis. 2015.10:195-262. Downloaded from www.annualreviews.org methods, that contain hollow microchannels inhabited by human cells; these devices recreate the specialized tissue-tissue interfaces, physicochemical microenvironments, and vascular perfusion characteristics of the lung, liver, gut, kidney, and many other living organs. We also describe how these organs-on-chips are being used to create novel in vitro models of human disease. STEM CELLS AND IN VITRO DISEASE MODELS The development of in vitro human disease models hinges on the availability of tissue- and organ- specific cell types that accurately recapitulate disease phenotypes. To date, most tissue engineering strategies rely on established cell lines (often transformed cell lines) or primary cells derived from patients with or without the disease of interest. Although primary cells are more representative of 196 Benam et al. PM10CH08-Ingber ARI 2 December 2014 10:14 the functional units of the tissue from which they are derived, they are difficult to obtain, proliferate slowly, have limited life span, and are often poorly characterized. Stem cells, particularly iPSCs (2), provide tremendous opportunities to overcome these limitations. Human iPSCs, which are derived from somatic cells by overexpression of a few transcription factors (3, 4), can be generated from patients with or without a specific disease, and the resulting pluripotent cells can self-renew indefinitely. Under appropriate conditions, these self-renewing human iPSCs can be differentiated into virtually any cell type. Although techniques for direct conversion of some differentiated cell types into other specialized cells (without reverting to the stem cell state) have also been reported (5–7), human iPSCs potentially offer an unlimited supply of cells for tissue engineering, therapeutic discovery, and modeling of diseases that affect almost all human tissues or organs. A growing number of reports have employed human iPSC-derived cells to model several diseases in vitro and, in some cases, aid drug testing and therapeutic discovery by illuminating disease mechanisms. Although these lines of investigation remain largely underexplored, a few reports have focused on uniting the principles of tissue engineering and stem cell biology to develop in vitro models of human disease, as we describe below. However, it is important to note that almost all specialized cell types derived by differentiation of iPSCs still exhibit immature phenotypes. Such immature cells may be relevant for studying early-onset disease processes, but it is less clear whether their biological responses can be extrapolated to mature and functional cell types that normally compose adult organs. As such, robust methods to not only differentiate iPSCs but also facilitate their maturation and expression of adult-like functionalities are needed. It is also less clear what combinations of soluble, insoluble, and mechanical signals are necessary to coax human iPSCs to commit to specific lineages. Given the importance of soluble, insoluble, and mechanical
Details
-
File Typepdf
-
Upload Time-
-
Content LanguagesEnglish
-
Upload UserAnonymous/Not logged-in
-
File Pages71 Page
-
File Size-