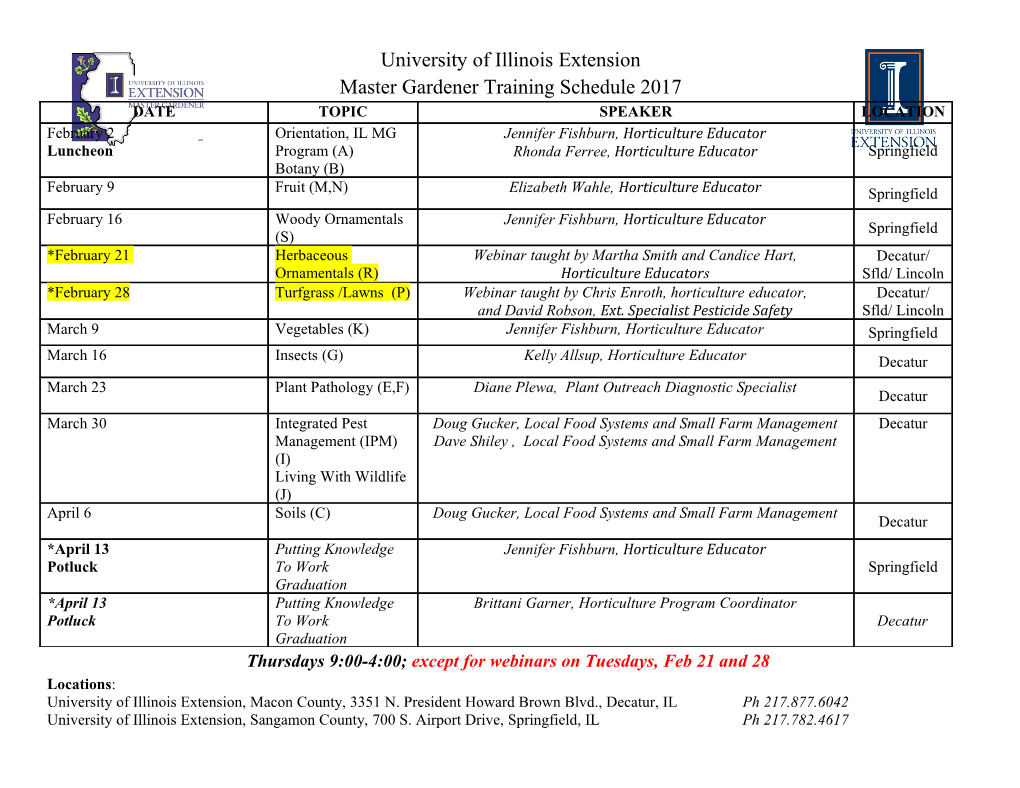
TECHNICAL GUIDE THE RADIOMETRY OF LIGHT EMITTING DIODES TABLE OF CONTENTS 1.0 Introduction . .1 2.0 What is an LED? . .1 2.1 Device Physics and Package Design . .1 2.2 Electrical Properties . .3 2.2.1 Operation at Constant Current . .3 2.2.2 Modulated or Multiplexed Operation . .3 2.2.3 Single-Shot Operation . .3 3.0 Optical Characteristics of LEDs . .3 3.1 Spectral Properties of Light Emitting Diodes . .3 3.2 Comparison of Photometers and Spectroradiometers . .5 3.3 Color and Dominant Wavelength . .6 3.4 Influence of Temperature on Radiation . .6 4.0 Radiometric and Photopic Measurements . .7 4.1 Luminous and Radiant Intensity . .7 4.2 CIE 127 . .9 4.3 Spatial Distribution Characteristics . .10 4.4 Luminous Flux and Radiant Flux . .11 5.0 Terminology . .12 5.1 Radiometric Quantities . .12 5.2 Photometric Quantities . .12 6.0 References . .13 1.0 INTRODUCTION Almost everyone is familiar with light-emitting diodes (LEDs) from their use as indicator lights and numeric displays on consumer electronic devices. The low output and lack of color options of LEDs limited the technology to these uses for some time. New LED materials and improved production processes have produced bright LEDs in colors throughout the visible spectrum, including white light. With efficacies greater than incandescent (and approaching that of fluorescent lamps) along with their durability, small size, and light weight, LEDs are finding their way into many new applications within the lighting community. These new applications have placed increasingly stringent demands on the optical characterization of LEDs, which serves as the fundamental baseline for product quality and product design. Characteristics of LEDs, including physical size, flux levels, spectrum and spatial distribution, separate them from typical element sources, which are generally employed and measured for photometric and radiometric quantities. With an LED, it is often difficult to achieve a high level of photometric or radiometric measurement accuracy due to uncertainties within the measurement equipment and improper test setup. In addition, traditional photometers, because of their inability to simulate the response of the human eye at the ends of the visible spectrum generate significantly flawed data when testing red, blue, and some styles of white LEDs. Specific expertise in LED metrology is needed in order to obtain accurate and reproducible results. This technical guide discusses the special optical characteristics of LEDs and provides recommendations for obtaining accurate measurement results. Our goal is to share our 20+ years of optical radiation measurement expertise and provide not only an introduction to LED metrology for individuals new to this subject area, but also a useful reference for individuals experienced in this area. 2.0 WHATISANLED? LEDs are solid-state (p-n junction semiconductor) devices that convert electrical energy directly into light (electroluminescence). LED "cold" generation of light leads to high efficiency because most of the energy radiates in the visible spectrum. In comparison, incandescent bulbs convert about 5 percent of their power into visible light, while LEDs approach 15 to 20 percent. Incandescent lamps radiate much of their energy in the non-visible spectrum, generating heat as well as light. For example, the package of an LED may be 10° to 25° C hotter than ambient, but under the same conditions, the envelope of an incandescent bulb can be several hundred degrees C hotter. 2.1 Device Physics and Package Design An LED in its simplest form is a semiconductor p-n junction device (chip) that, when forward biased, emits photons (light) as the electrons and holes recombine near the junction. The energy of the photons is determined primarily by the energy bandgap of the semiconduc- tor where the recombination occurs. Since the eye is only sensitive to light with photon energy from 3.1 eV to 1.6 eV (0.40 to 0.78 µm), compound semiconductor materials composed of column III and V elements are the materials of choice for LEDs because they have the direct bandgap properties and energies necessary for efficiently producing visible photons. To convert the wavelength (λ) in microns to photon energy, the relationship λ = 1.24 / eV can be used. Figure 2.0 shows the semiconductors of interest with their corresponding photon energies, wavelengths, and the relative response of the human eye. 1 The energy gap of a semiconductor is the minimum energy separating the valence band and the conduction band. Each band contains the possible combinations of energy and momentum for one type of carrier — the valence band for the carriers of positive charge (holes) and the conduction band for negative charge carriers (electrons). An LED's internal quantum efficiency is the number of photons generated divided by the number of minority carriers (electrons) injected into the p-doped region. When an injected electron combines with a hole through radiative recombination, a photon is produced. There are other kinds of transitions that compete with radiative recombination, but these transitions fail to produce photons. These transitions occur at crystal imperfections of various sorts and dissipate their energy into the crystal lattice as heat. For those photons that are generated, there can still be loss through absorption within the LED material, reflection loss when light passes from a semiconductor to air due to differences in refractive index and total internal reflection of light at angles greater than the critical angle defined by Snell's law, lowering the overall quantum efficiency of the LED. Fig. 2.0. Figure 2.1 represents a basic LED chip structure with an opaque or a transparent substrate. Semiconductors of interest as visible LEDs. Figure includes The light-generating LED chip is quite small, typically 0.25 millimeters square. Figure 2.2 relative response of human eye depicts a diagram of a typical LED lamp. An LED lamp contains an LED chip and an epoxy molded lens encapsulate. The lens is used to change the direction and control the distribu- tion of light rays (spatial distribution pattern) or colored to serve as an optical filter to enhance contrast. The epoxy encapsulant and lead frame occupy most of the volume. Presently the most common LED is the 5mm LED package (or T 1-3/4). Fig 2.1. Basic LED Chip Structure COLORED EPOXY LENS GLASS WINDOW LED CHIP METAL CAN METAL HEADER GLASS INSULATOR CATHODE ANODE (-) (+) Fig 2.2. LED Lamp Construction (a) 2 2.2 Electrical Properties 2.2.1 Operation at Constant Current The drive current through an LED must be controlled. High current densities within the junction of the chip cause partial overheating which damages the crystalline structure of the LED die. At these areas are so called darkline defects, where light ceases to be generated. This should not be confused with the maximum junction temperature of the LED, which is related to the higher temperature at which the optical grade epoxy starts to expand rapidly and increases the risk of catastrophic failures, such as broken wires or lifted LED dies. To produce light, an LED must be operated in the forward-bias regime. The emitted light is a function of the forward voltage Vf and the forward current If. In the lab, LEDs are usually operated in a forward bias direction from a constant current DC power supply. At low currents, the slope of the radiant power (luminous flux) verses time rises faster than the slope of the electrical power (start-up range) verses time. At high currents, the slope becomes flatter (saturation area), which is mainly caused by heating of the LED chip. Under normal operating conditions (between the start-up range and saturation area), the optical radiation emitted by LEDs is strongly correlated to the electrical current, which is why constant current is recommended for measurements intended to characterize the optical properties of an LED. 2.2.2 Modulated or Multiplexed Operation One efficient method of driving LED devices is to pulse them (usually 100Hz to 1000Hz) with a high peak current for short durations on a low duty factor. This technique is frequently used to multiplex a number of individual LED lamps. The advantage is high light output at low time average power consumption. LED operation under these non-steady-state conditions can create temperature fluctuations at the junction and forward voltage instability, which modifies the characteristic values obtained for the LED under test. Fortunately, these values are strongly correlated to the values for steady-state operations, so that the true characteristics of the LED under test can be calculated once the correlation is derived. 2.2.3 Single-Shot Operation During production, the measurements commonly made to characterize an LED are often carried out under single-shot operations where the LED under test is pulsed with a DC current equaling that of normal operations but only lasting tens of milliseconds. Similar to modulated operations, the LED fails to reach steady-state conditions, but the correlation between single-shot and steady-state conditions can be established by a few supplementary measurements. 3.0 OPTICAL CHARACTERISTICS OF LEDS The radiation from an LED can be characterized by radiometric and spectroradiometric quantities. If the LED emits visible radiation, then photometric and colorimetric quantities are also required to quantify its effect on the human eye. Note that for every radiometric quantity there is a photometric analog. The only difference is that, for radiometric quantities the radiation is evaluated in energy units, while for photometric quantities the radiation is weighted against the photopic response of the human eye. 3.1 Spectral Properties of Light Emitting Diodes The spectral distribution of the optical radiation emitted by LEDs distinguish them from typical element sources. The radiant power is neither monochromatic (as emitted by lasers), nor broadband (as found with incandescent lamps), but rather something between the two.
Details
-
File Typepdf
-
Upload Time-
-
Content LanguagesEnglish
-
Upload UserAnonymous/Not logged-in
-
File Pages18 Page
-
File Size-