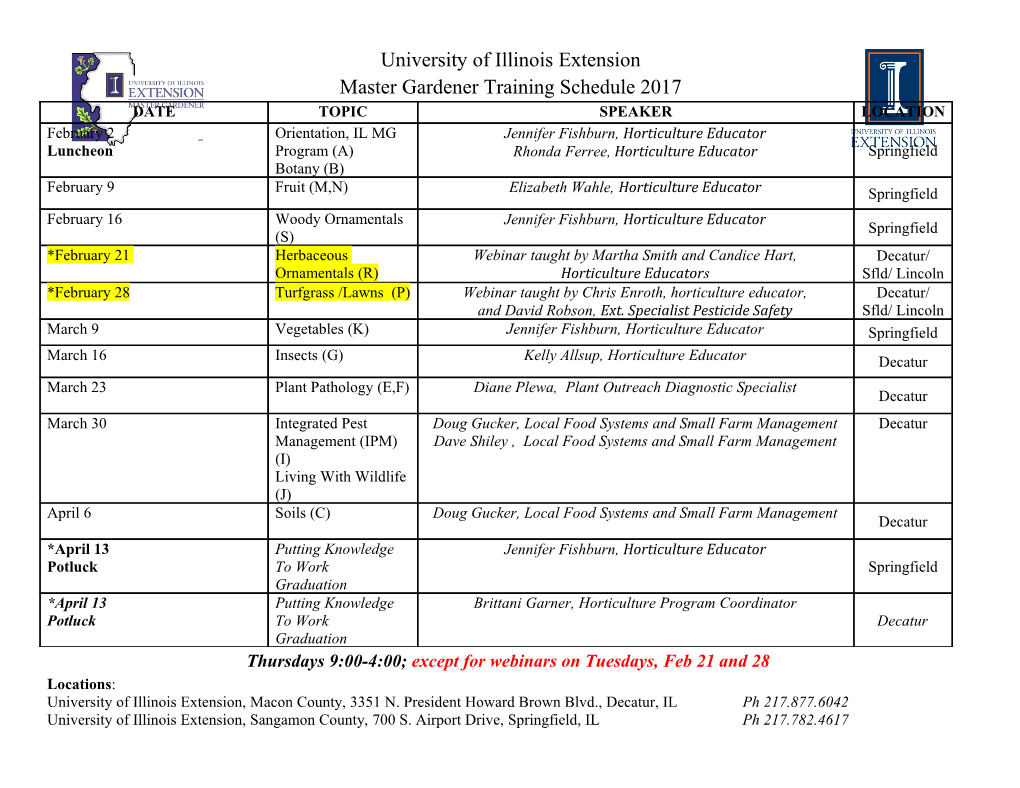
CELLULAR NEUROPHYSIOLOGY CONSTANCE HAMMOND Chapter 1 Zoom in on… Patch configurations In the jargon of electrophysiologists, a patch is a piece of neuronal membrane. Researchers invented a technique known as a patch-clamp, which records the current through a single ion channel, some ion channels or through all open ion channels in the neuron membrane. To obtain these recordings, researchers use different patch configurations. We'll explain here only the three configurations used in the course: the cell-attached configuration, the whole-cell configuration, and the "outside-out" excised patch configuration. According to the type of recording to perform, a particular type of configuration will be chosen: 1. to record a unitary current: cell-attached or outside-out configuration; 2. to record a total current: whole-cell configuration; 3. to record changes in membrane potential (action potentials or postsynaptic potentials): whole-cell configuration. The cell-attached configuration (attached to the pipette - Figure a) First, the pipette is filled with an extracellular fluid. Positive pressure is applied in the electrode by means of a syringe connected to the electrode, so that the intrapipette fluid tends to leave the pipette. The electrode is then brought near to the soma of a neuron. When the electrode touches the membrane, the positive pressure is withdrawn to draw the membrane toward the mouth of the pipette. We wait without moving the pipette; a seal is made between the walls of the pipette and the soma membrane. This seal strength can be measured by applying a low level of amplitude current in the pipette. Since V = RI, one can measure the resistance of the seal. If a step ΔV (in mV) is applied through the pipette, the recorded response is a change in the current ΔI (in pA), such that ΔV = R ΔI. This allows us to calculate R (in GΩ), the seal resistance. In order for the seal to be perfect it is necessary that the resistance of the seal is on the order of gigaohms (109 Ohms = 1 GΩ). Thus, the neuron membrane is sealed to the mouth of the pipette. The pipette can be placed at the soma and also at a dendrite. The cell-attached configuration in voltage-clamp mode records the unit current through one or more open channels in the patch of membrane underneath the pipette mouth. The intracellular medium of the neuron is its physiological medium. Recording a total current: whole-cell configuration (Figure b) The whole-cell configuration means that the recording electrode is continuous with the entire membrane of the neuron. To accomplish this, the pipette is filled with an artificial intracellular solution that will become the intracellular medium of the neuron (i). We start from a cell- attached configuration with a seal on the order of GΩ. Then, suction is applied to the interior of the pipette (negative pressure) for attracting the small piece of membrane which plugs the opening of the pipette towards the interior of the pipette. We know that this piece of membrane has been removed from the pipette opening because the access resistance is much lower (the pipette is no longer plugged). In the whole-cell configuration, the intrapipette solution gradually replaces the intracellular medium. This voltage-clamp mode configuration records the current passing through all open ion channels in the neuronal membrane, i.e. a total current is recorded. In current-clamp mode, this measures changes in potential across the entire neuronal membrane, i.e. action potentials or postsynaptic potentials are recorded (seen in upcoming chapters). The pipette can be placed at the soma, and also at a dendrite. The outside-out configuration (Figure c) The outside-out configuration means that the recording electrode records the activity of a small piece of membrane whose external side is in contact with the artificial extracellular solution. When we want to record a channel situated in a piece of somatic membrane, everything begins exactly as for a whole-cell configuration. Once this configuration is obtained, the pipette is gently lifted in order to stretch the membrane of the soma (Figure c). At one point a piece will detach and close in on itself. We know that the excised patch configuration has been achieved because the membrane resistance becomes very low. An outside-out patch can also be obtained at the dendritic membrane. This configuration records the unitary current traversing a single channel in voltage-clamp mode. Compared to the cell- attached configuration, the outside-out configuration enables researchers to change the MOOC Cellular neurophysiology – Ch.1 – Zoom – 2/8 intracellular medium of the neuron and to easily add pharmacological agents into the extracellular medium. In summary In both whole-cell and outside-out configurations, the intracellular medium is the intrapipette environment and the extracellular environment is the bath medium. Neither of the two media is the actual medium of the recorded neurons. In the cell-attached configuration, the intracellular medium remains intact and the extracellular medium is the bath medium. Voltage-clamp/current-clamp There are two major recording modes for neuronal activity: voltage-clamp mode and current- clamp mode. The experimenter chooses one or the other mode depending on what he wants to measure: measurement of current in voltage-clamp mode, and measurement of the changes in potential in current-clamp mode. In voltage-clamp mode, the total membrane potential (whole-cell configuration) or a small piece of membrane (cell-attached or outside-out configurations) is kept constant at a value set by the experimenter, called VH (V for Potential and H for Hold ). To maintain this potential, during the recording, any change in the recorded value from the set VH value is immediately compensated by the injection of a positive or negative current through the recording electrode. It's like constantly adding more water into a leaky vessel so that the water level stays the same. The voltage-clamp mode measures I (current across the entire neuronal membrane) or i (current crossing an ion channel), depending on the selected patch configuration. Changes in I or i will then depend only on G or γ (total or unitary conductance) according to the formula I = GVH, where i = γV H. In current-clamp mode, the experimenter measures variations in the membrane potential. In this mode, the value of the current injected through the electrode is selected by the experimenter. Depolarization, hyperpolarization, repolarization The reference point for changes in membrane potential is often the resting potential (in current-clamp recordings) or the holding membrane potential (in voltage-clamp recordings). A depolarization is a change in potential towards potentials less hyperpolarized than the resting or holding membrane potential. For example, if the membrane changes from -60 to - 40 mV, it is said to depolarize. MOOC Cellular neurophysiology – Ch.1 – Zoom – 3/8 It also depolarizes if it changes from -60 mV to +20 mV. A hyperpolarization is a change in potential towards potentials more hyperpolarized than the resting or holding membrane potential. For example, if the membrane changes from -60 to - 80 mV, it is said to hyperpolarize. A repolarization is a return to the resting or holding membrane potential. From a depolarized value, the membrane gets repolarized by hyperpolarizing. From a hyperpolarized value, the membrane gets repolarized by depolarizing. The Nernst equation gives the equilibrium potential of an ion The direction of the passive transport of an ion species depends on its concentration on both sides of the membrane: ions move passively from the medium where they are more concentrated towards the medium where they are less concentrated. Because ions are charged molecules, their direction of passive transport through the membrane also depends on the membrane potential (Vm = Vi – Vo): negatively-charged ions are attracted to the + side of the membrane and vice versa. When ion concentrations are constant, the only variable is the membrane potential, which changes depending on the signals received by the neuron. Thus, the passage of an ion can change direction, depending on Vm. Take Na+ ions from the above diagram as an example. MOOC Cellular neurophysiology – Ch.1 – Zoom – 4/8 Loot at their concentration gradient (red arrow): the concentration of Na+ ions is higher outside than inside. Thus, Na+ ions tend to enter the neuron through the open Na+ channels. Now consider their electrical gradient (green arrow): the internal side of the membrane is more negatively charged than the external side. This also attracts the Na+ ions inside the neuron. Overall, the net flux of Na+ ions is therefore inward (blue arrow): positively-charged Na+ ions tend to move inside the neuron. + But when Vm is reversed during the action potential, what are the Na ions doing? In the second diagram, we see that, while the magnitude of the concentration gradient remains the same, the direction of the electrical gradient has reversed. How does it then affect the flow of Na+ ions? To solve this problem, we must quantify the above situation. The Nernst equation gives the equilibrium potential of an ion (Eion). The definition of equilibrium potential is as follows: when Vm = Eion, the net flow of this ion is zero. When Vm = Eion, this ion is in equilibrium. It is around this Eion equilibrium potential that the net flow of this ion is reversed. where: R is the gas constant, T is the absolute temperature in K°, z is the valence of the ion, F is Faraday's constant. In other words: MOOC Cellular neurophysiology – Ch.1 – Zoom – 5/8 + For Na ions, at the given concentrations, ENa = 58/1 log(140/14) = +58 mV + As long as Vm is more hyperpolarized than +58 mV, Na ions passively enter the neurons + + through the open Na channels.
Details
-
File Typepdf
-
Upload Time-
-
Content LanguagesEnglish
-
Upload UserAnonymous/Not logged-in
-
File Pages8 Page
-
File Size-