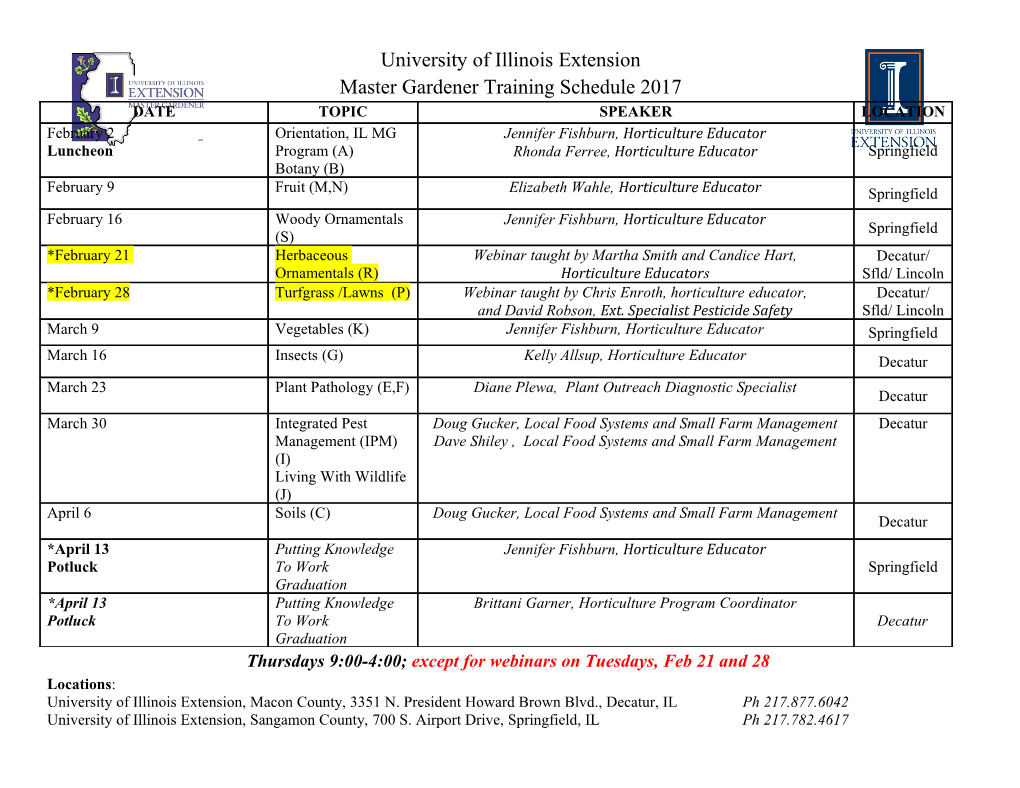
Supplementary Information Enhanced limonene production in cyanobacteria reveals photosynthesis limitations Xin Wang, Wei Liu, Changpeng Xin, Yi Zheng, Yanbing Cheng, Su Sun, Runze Li, Xin-Guang Zhu, Susie Y. Dai, Peter M. Rentzepis1 and Joshua S. Yuan1 1To whom the correspondence should be addressed Peter M. Rentzepis: [email protected] (979)845-7250 Joshua S. Yuan: [email protected] (979)845-3016 Table of Contents: Supplementary Methods Supplementary Figure 1-6 Supplementary Dataset 1 Supplementary Table 1-3 Supplementary Files 1-4 References 1 Supplementary Methods Strains and plasmids construction. Strains and plasmids used in this study were summarized in Table S1. The maps and sequences of pWX1118 and pWX121 can be found in File S2 and S3. Plasmids were constructed using Gibson Assembly (NEB, Ipswich, MA) and primers used were designed using NEBuilder Assembly Tool (http://nebuilder.neb.com/). Limonene synthase (LS) was codon optimized for S. elongatus expression and synthesized by IDT (Coralville, IA). S. elongatus genome neutral site I targeting plasmids were constructed based on pAM2991 (from Golden lab), and neutral site II targeting plasmids were constructed based on pAM1579 (from Addgene). Cell absorbance spectra scan and optical density 200 µl of wild type or limonene-producing cells were used to measure the whole-cell absorbance wavelength scans using an Epoch Microplate Spectrophotometer (BioTek Instruments Inc., Vermont). The absorbance scans were normalized by cell density (OD730). All measurements were determined by averaging triplicates of independent cultures. Error bars in figures represent standard deviations. Model description and simulations. A kinetics model of MEP terpene biosynthesis was developed by extending the C3 photosynthesis kinetics model developed by Xin et al. (1). The current model was built with Simbiology in MATLAB (version 2008a). The sundials solver of Simbiology was chosen to solve the system. The solution of ODEs provided by sundials was the time evolution of the concentration of each metabolite in each compartment which in turn allows calculation of different reaction fluxes. In our model, the carbon input into terpene synthesis was channeled from photorespiratory pathways through malate synthase. The glyoxylate was first converted to malate, and further to pyruvate by malate dehydrogenase (Fig. S6). All pyruvate channeled from photorespiration was used for terpene synthesis through a simplified MEP derived terpene biosynthesis pathway (Fig. S6). The initial concentrations of metabolites and the kinetic parameters of the additional reactions used in this model were listed in the supplemental tables (Tables S2 and S3). The reaction rates of the additional enzymes were described using the Michaelis-Menten kinetics (File S4). In order to control carbon flux for terpene synthesis, we used glycolate dehydrogenase to control carbon input, and assumed that the pyruvate used for MEP pathway was only generated from photorespiration. By changing Vmax of glycolate dehydrogenase, the carbon flux into MEP pathway could be manipulated in the simulations. Protein preparation for proteomics. S. elongatus wild type and limonene producing lines were grown to log phase in 1 L Roux culture bottles as described above. 100 mL of each line was collected in triplicates, and centrifuged at 8000 rpm for 15 min at 4 °C. Cell pellets were resuspended in 2 mL Tris buffer (50 mM Tris- TM HCl, 10 mM CaCl2, 0.1 % Nonidet P-40, pH 7.6) supplemented with 1X Halt protease and phosphatase inhibitor cocktail (Thermo Scientific, Rockford, IL). Cells were lysed through 10 cycles of sonication with 30 seconds on and 2 min off on ice, and centrifuged at 13,000 g for 30 min at 4 °C. The supernatant was collected and transferred into a new centrifuge tube. Protein concentration was determined by Bradford assay (Thermo Scientific, Rockford, IL) following the manufactory’s instructions. Liquid chromatography-tandem mass spectrometry (LC-MS/MS). The Multidimensional Protein Identification Technology (MudPIT) based shotgun proteomics were performed similarly as Washburn et al. (2). For each sample, 100 μg of total protein was first 2 incubated with 8 M urea and 5 mM dithiothreitol (DTT) at 37 °C for an hour. The protein was then diluted 4 folds using Tris buffer (50 mM Tris-HCl, 10 mM CaCl2, pH 7.6), and subjected to trypsin digestion using Mass Spectrometry Grade Trypsin Gold (Promega, Madison, WI) with 1:100 w/w at 37 °C for 18 hours. The digested peptides were cleaned up using a Sep-Pak C18 plus desalting column (Waters Corporation, Milford, MA). A biphasic strong cation exchange/reversed phase capillary column was prepared to load the peptides with a pressure cell, followed by a two dimensional peptide separation as described previously (3). Peptide fractions were analyzed on a LTQ ion trap mass spectrometer (Thermo Finnegan, San Jose, CA) operated in the data-dependent acquisition mode. An MS2 method was used to record the full mass spectra over the range of 300- 1700 m/z. The 5 most abundant peaks were subjected to collision induced dissociation fragmentation for MS/MS analysis. The proteomics analyses were carried out with triplicate biological samples and consolidated in the final data analysis. Database search and spectral validation. A data processing pipeline was developed to analyze the peptide information. MS2 file of tandem mass spectra was first extracted from the raw data, and subsequently used for database search by ProLuCID (4) (version 1.0). A protein database were generated including S. elongatus proteins (2657 sequences) obtained from UniProt database and 37 common contaminants, and their reversed sequences (total 5388 sequences). The reverse database was included as a quality control system to restrain false positive discovery to 0.05. ProLuCID search was performed using a precursor mass tolerance of 100 ppm. And no peptide modifications were included in the search. The peptide/spectrum matches were filtered using DTASelect (5) (v. 2.0) with the criteria of DelCN ≥ 0.08 coupled with XCorr ≥ 1.8 for charge state +1, ≥ 2.5 for charge state +2, and ≥ 3.5 for charge state +3. A minimum of two peptides were needed to identify proteins. Analysis of protein differential expression. Protein differential expression was analyzed in PatternLab (6) (version 4.0.0.38) with spectral counts indicating protein abundance. The normalized spectral abundance factor (NSAF) (7) was applied to the biological triplicates to minimize the noise caused by protein length and sample-to- sample variation. TFold test was used to pinpoint the protein differential expression, in which the BH q-value and L-stringency were set to 0.05. Variable fold change was determined by the optimized F-stringency parameter. 3 Fig. S1. Limonene production in S. elongatus through stepwise metabolic engineering. (A) MEP pathway for IPP/DMAPP generation. S. elongatus does not encode a limonene synthase (LS) or geranyl pyrophosphate synthase (GPPS). Heterologous LS was introduced into S. elongatus genome to enable limonene synthesis. (B) Specific limonene productivity in different strains. Strain L111: ls driven by Ptrc promoter and integrated in neutral site I of S. elongatus genome; Strain L113: ls driven by Ptrc promoter in which the native RBS was replaced by a synthetic RBS sequence; L114: ls/gpps in a single operon driven by Ptrc promoter; L114/dxs: dxs driven by PlacO-1 promoter and integrated in neutral site II of strain L114 genome. 4 Fig. S2. GC-MS analysis of limonene production in engineered cyanobacteria. Figure represents a typical elution from the hydrocarbon absorption trap with cedrene as the internal standard. 5 Fig. S3. Normalized spectral count for limonene synthase in L1115 and L1118 cells. Normalized spectral count was calculated by dividing peptide spectral counts with the protein length of limonene synthase (543 amino acids). Data was obtained from biological triplicates with error bars indicating standard deviations. The two-tailed t-test showed a significant difference between L1115 and L1118 LS levels (p value = 0.0005). 6 Fig. S4. Cell growth and limonene productivity of L1118. (A) Growth of wild type and L1118 cells. (B) Daily limonene productivity in L1118 cells. Error bars indicate standard deviations. One- way ANOVA (p < 0.0001) showed that limonene production is significantly different in at least two time points. 7 Fig. S5. Standard curve used to calculate limonene recovery rate. Limonene concentrations of 10, 50, 100, and 250 µg/mL were used to spike the S. elongatus wild type cells. Limonene was collected and measured as described in the methods. 8 Fig. S6. Pathway in kinetics modeling. The current model is an expansion of a previous C3 photosynthesis kinetics model (1). Carbon flux into MEP derived terpene biosynthesis was controlled by pyruvate generation through glycolate dehydrogenase in photorespiration. A simplified downstream terpene biosynthesis pathways were used to simulate downstream terpene response against MEP carbon flux. 9 Dataset S1. Differential expression of proteins in WT and L1118 cells. The statistical analysis of changes in protein expression was conducted by PatternLab (see methods), and ranked by protein fold change in the dataset. Each row is a separate protein, and the columns from left to right show the UniProt ID of the protein, fold change between WT and L1118 proteins, p-value calculated from PatternLab showing the statistical difference between WT and L1118 proteins, the normalized spectral
Details
-
File Typepdf
-
Upload Time-
-
Content LanguagesEnglish
-
Upload UserAnonymous/Not logged-in
-
File Pages23 Page
-
File Size-