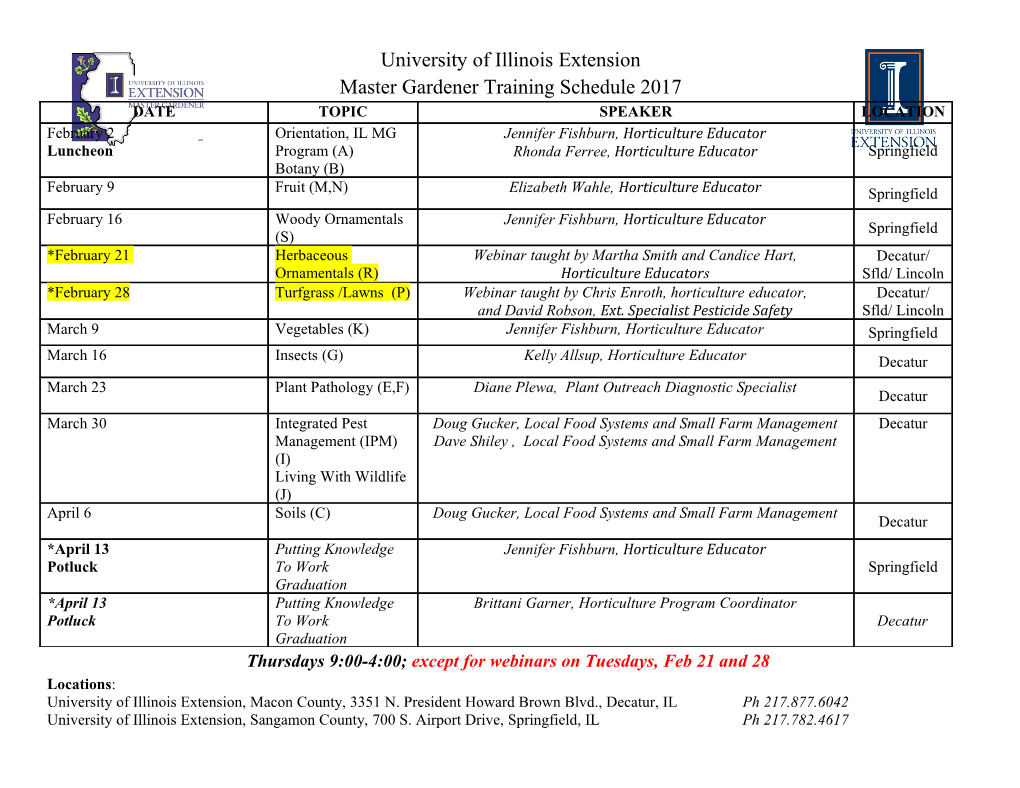
tBu C 3 Rh tBu CH Hydrogen Atom + N 3 Transfer THF, rt 3 -1 -1 kH• = 1.2 × 10 M s FAST! BDFE = 52.3 kcal/mol BDFE ~ 70 kcal/mol tBu N P N N N tBu H H Proton N Transfer Rh Rh P N N N + N N CD3CN, rt -4 -1 -1 kH+ = 5 × 10 M s Rh(III)–H SLOW! pKa = 30.3 pKa = 28.4 N BF4 Me NCCH3 Hydride N Rh BF4 + Transfer N Me CH3CN, rt 5 -1 -1 kH- = 3.5 × 10 M s FAST! ∆GºH- = 49.5 kcal/mol ∆GºH- > 90 kcal/mol Hu, Y.; Norton, J. R., J. Am. Chem. Soc. 2014, 136, 5938-5948. Thermochemistry of Transition Metal Hydrides: Bond Strength, Acidity, and Hydricity Nick Shin The Knowles Group Princeton University Group Meeting September 28, 2019 Hieber, W.; Leutert, F., Naturwissenschaften 1931, 19, 360-361. Outline 1. Brief Overview of Metal Hydrides 2. Bond Strength of Transition Metal Hydrides 3. Acidity of Transition Metal Hydrides 4. Hydricity of Transition Metal Hydrides Types of Metal Hydrides What is a Hydride? • The anion of hydrogen, H- • A compound in which one or more hydrogen centers have nucleophilic, reducing, or basic properties 1. Ionic Hydrides • Hydrides bound to alkali metal or alkaline earth metal • NaH, KH, CaH2, … • Applications: strong base, reducing reagent, dessicant. CaH2 2. Interstitial (Metallic) Hydrides • Hydrides within metals or alloys • Non-stoichiometric adsorption of H atoms in the lattice • Examples: Ni–H for nickel-metal hydride battery (NiMH), Pd–H for fuel cells, heterogenous catalysis Mcgrady, G. S.; Guilera, G., Chem. Soc. Rev. 2003, 32, 383-392. Types of Metal Hydrides 3. Molecular (Covalent) Hydrides • All other metal hydrides • p-block elements: common reducing reagents “Superhydride” NaBH4 LiAlH4 DIBAL TTMS • and… transition metal hydrides! Re H The first molecular hydride The first “organometallic TM hydride” Hieber (1931) Wilkinson (1955) Mcgrady, G. S.; Guilera, G., Chem. Soc. Rev. 2003, 32, 383-392. Norton, J. R.; Sowa, J., Chem. Rev. 2016, 116, 8315-8317. Wilkinson, G.; Birmingham, J. M., J. Am. Chem. Soc. 1955, 77, 3421-3422. Transition Metal Hydrides in Catalysis Hydrogenation (Food, Petrochemical, Pharmaceutical, and Agrochemical Industries) MeO MeO MeO Ir/JosiPhos O 50 ºC, H2 (80 bar) Cl Me N Me NH Me N Me yield > 90% Me Me Me Me Me ee = 80% TON = 2,000,000 TOF = 400,000 h–1 (S)-metolachlor Herbicide (Dual Magnum®) > 10,000 t / year Hydroformylation (Detergent, Fragrance, Petrochemical, and Pharmaceutical Industries) H O H O HCo(CO)4, H2, CO Production Scale: R + H 6.6 million tons (1995) R H R 120–180 ºC, 200–300 atm 10.4 million tons (2008) 3-4 : 1 Chemical Processes: Hydrosilylation, hydrodesulfurization, C–H functionalization, olefin isomerization Energy conversion: CO2 reduction, H2 oxidation, reversible H2 storage and more H. U. Blaser, F. Spindler and M. Studer, App. Cat. A: Gen. 2001, 221, 119-143. Hartwig, J. F., Organotransition Metal Chemistry: From Bonding to Catalysis, University Science Books, 2010. Versatile Reactivity of Transition Metal Hydrides The three thermochemical properties: pKa, BDFE, and ∆GºH- What is covered: • Definition • Experimental methods for measurement of each thermochemical property • General trends with a focus on late transition metals • Relevant examples in catalysis Today’s goal: Have a ballpark figure for acidity, BDFE, and hydricity of any transition metal hydride complex Wiedner, E. S.; Chambers, M. B.; Pitman, C. L.; Bullock, R. M.; Miller, A. J. M.; Appel, A. M., Chem. Rev. 2016, 116, 8655-8692. Bond Strength of Transition Metal Hydrides Definition LnM–H LnM • + H • ∆Gº = Bond Dissociation Free Energy (BDFE) Experimental Method of Determining M–H BDFE: Thermochemical Cycle BDFE (M–H) LnM–H LnM• + H• 2.3 RT pKa(M–H) 0.5 BDFE (H2) – + LnM + H LnM• + 0.5 H2 – + F [Eº(M /M) - Eº(H /H2)] – – BDFE (M–H) = 1.37 pKa + 23.06 Eº(M /M) + 53.6 kcal/mol Measure pKa (explained later) & Eº(M /M) (via CV) (CH3CN, rt) Other methods include calorimetric measurement and kinetic measurement using FT-ICR-MS, but those require well-understood decomposition pathways. FT-ICR-MS (left), “the most complex method of mass analysis and detection.” Tilset, M.; Parker, V. D., J. Am. Chem. Soc. 1989, 111, 6711-6717. Mass Spectrometer Facility at the University of Bristol (accessed September 24, 2019). http://www.chm.bris.ac.uk/ms/ Trends in M–H Bond Strength 1. Bond Dissociation Enthalpy (gas) ~ Bond Dissociation Free Energy (sol) + 4.8 kcal/mol LnM–H (g) LnM • (g) + H • (g) ∆Hº = BDE (M–H)gas LnM–H (sol) LnM • (sol) + H • (sol) ∆Gº = BDFE (M–H)sol 2. Most metal hydrides have M–H BDFE’s between 55 and 65 kcal/mol. 3. M–H bond strengths: first < second < third row 4. Electronic properties of ligands have little effect on the M–H bond strength. ← actually BDFE in our definition Wayner, D. D. M.; Parker, V. D., Acc. Chem. Res. 1993, 26, 287-294. Tilset, M.; Parker, V. D., J. Am. Chem. Soc. 1989, 111, 6711-6717. Simoes, J. a. M.; Beauchamp, J. L., Chem. Rev. 1990, 90, 629-688. Trends in M–H Bond Strength 4. BDE (M–H) is almost always greater than BDE (M–C). 5. Rates of H• transfer are substantially influenced by sterics and bond strength. M–H + tBu CH M • + tBu C 3 3 BDE ~ 75 kcal/mol Simoes, J. a. M.; Beauchamp, J. L., Chem. Rev. 1990, 90, 629-688. Eisenberg, D. C.; Lawrie, C. J. C.; Moody, A. E.; Norton, J. R., J. Am. Chem. Soc. 1991, 113, 4888-4895. Selected Example: Radical Hydrofunctionalization of Olefins Initial Mechanistic Observation: Halpern (1975) R HCo(CO)4 H R HCo(CO)4 H R R Co(CO)4 R Co(CO)4 R H 2 Co(CO)4 Co2(CO)4 Observations: 1) When using DCo(CO)4, H/D exchange at 9/10 positions is faster than hydrogenation. 2) Introduction of alkyl groups R increases the rate. 3) Equal mixture of cis-/trans-hydrogenation. H• Addition to Olefin: Halpern (1977) HMn(CO) • Mn(CO)4 HMn(CO) 4 Me 4 Me Ph Ph Me Ph Me • Mn(CO)4 Ph Me Me Me • Mn(CO) radical cage 4 Observations: 1) 1st order kinetics in both alkene and hydride. 2) First HAT is reversible. 3) When reacted with DMn(CO)4, D incorporation in both reactant and product. 4) Inversie isotope effect of 0.4 observed with H/DMn(CO)4. Feder, H. M.; Halpern, J., J. Am. Chem. Soc. 1975, 97, 7186-7188. Sweany, R. L.; Halpern, J., J. Am. Chem. Soc. 1977, 99, 8335-8337. Selected Example: Radical Hydrofunctionalization of Olefins Challenge: Thermodynamically Unfavorable H• Addition Me Ph • Mn(CO)4 H BDFE(βC–H) ~ 35–45 kcal/mol Me + H–Mn(CO)4 Ph BDFE(M–H) ~ 55–65 kcal/mol Weak M–H bond & stable product radical required. Tang, L.; Papish, E. T.; Abramo, G. P.; Norton, J. R.; Baik, M.-H.; Friesner, R. A.; Rappé, A., J. Am. Chem. Soc. 2003, 125, 10093-10102. Selected Example: Radical Hydrofunctionalization of Olefins Tang, L.; Papish, E. T.; Abramo, G. P.; Norton, J. R.; Baik, M.-H.; Friesner, R. A.; Rappé, A., J. Am. Chem. Soc. 2003, 125, 10093-10102. Selected Example: Radical Hydrofunctionalization of Olefins Huge Advantage: Better Chemoselectivity Compared to Bronsted Acid Reactions Initial Inspiration from Biology General Scheme for Reductive-Oxidative Cycle Toward Alkene Hydrofunctionalization Crossley, S. W. M.; Obradors, C.; Martinez, R. M.; Shenvi, R. A., Chem. Rev. 2016, 116, 8912-9000. Selected Example: Mukaiyama Hydration Isayama and Mukaiyama of Mitsui Petrochemical Industries (1989): Co(acac) (20 mol%) 2 O OH O2 Ph Ph Me + Ph Me + Ph Me iPrOH (0.2 M) 8% 46% 17% 75 ºC, 1 hr. Mukaiyama’s Proposed Mechanism: Nojima (2002): II OOSiEt3 L2Co II iPrOH L2Co O2 R Me R L CoIII–H Et3SiH 2 III L2Co –O• III L2Co –OO• -2.8 ppm OH III 1 Co L2 ( H NMR) L CoIII O 2 R Me O iPrOH R Me III H Co L2 R O O III L CoII L2Co –OOH II OO 2 H L2Co R R Me R Me R O2 Note: Halpern and Norton’s reports suggest HAT instead of hydrometalation Teruaki, M.; Shigeru, I.; Satoshi, I.; Koji, K.; Tohru, Y.; Toshihiro, T., Chem. Lett. 1989, 18, 449-452. Tokuyasu, T.; Kunikawa, S.; Masuyama, A.; Nojima, M., Org. Lett. 2002, 4, 3595-3598. Crossley, S. W. M.; Obradors, C.; Martinez, R. M.; Shenvi, R. A., Chem. Rev. 2016, 116, 8912-9000. Selected Example: Dualcatalytic Hydrofunctionalizaiton Hydroalkylation (Shenvi, 2019): O O Mn(dpm)3 (20 mol%), Ni(acac)2 (2.5 mol%) O O PhSiH3 (3 equiv), HFIP (2 equiv) PMBO Me PMBO + Me tBu tBu I TBSO K2CO3 (1 equiv) TBSO Me DCE:Propylene Carbonate = 9:1 dpm rt, air 77% … and many substrates Proposed Mechanism: Green, S. A.; Huffman, T. R.; Mccourt, R. O.; Van Der Puyl, V.; Shenvi, R. A., J. Am. Chem. Soc. 2019, 141, 7709-7714. Selected Example: Dualcatalytic Hydrofunctionalizaiton Electrocatalytic Enantioselective Hydrocyanation (Lin, 2019): Proposed Mechanism: [Co] (0.5 mol%) CN Cu(OTf) (5 mol%) 2 H L (10 mol%) tBu tBu PhSiH3 (1.1 equiv) TMSCN (2.0 equiv) 79%, 91% ee TBABF4 (2.0 equiv) HOAc (5.0 equiv) DMF, 0 ºC, 10 h C(+)/Pt(–), Ucell = 2.3 V N N O O Co N N tBu O O tBu O O OtBu tBuO tBu tBu [Co] L … and many substrates Lu, S.; Niankai, F.; Brian G., E.; Wai-Hang, L.; Michael O., F.; Robert A., D.
Details
-
File Typepdf
-
Upload Time-
-
Content LanguagesEnglish
-
Upload UserAnonymous/Not logged-in
-
File Pages38 Page
-
File Size-