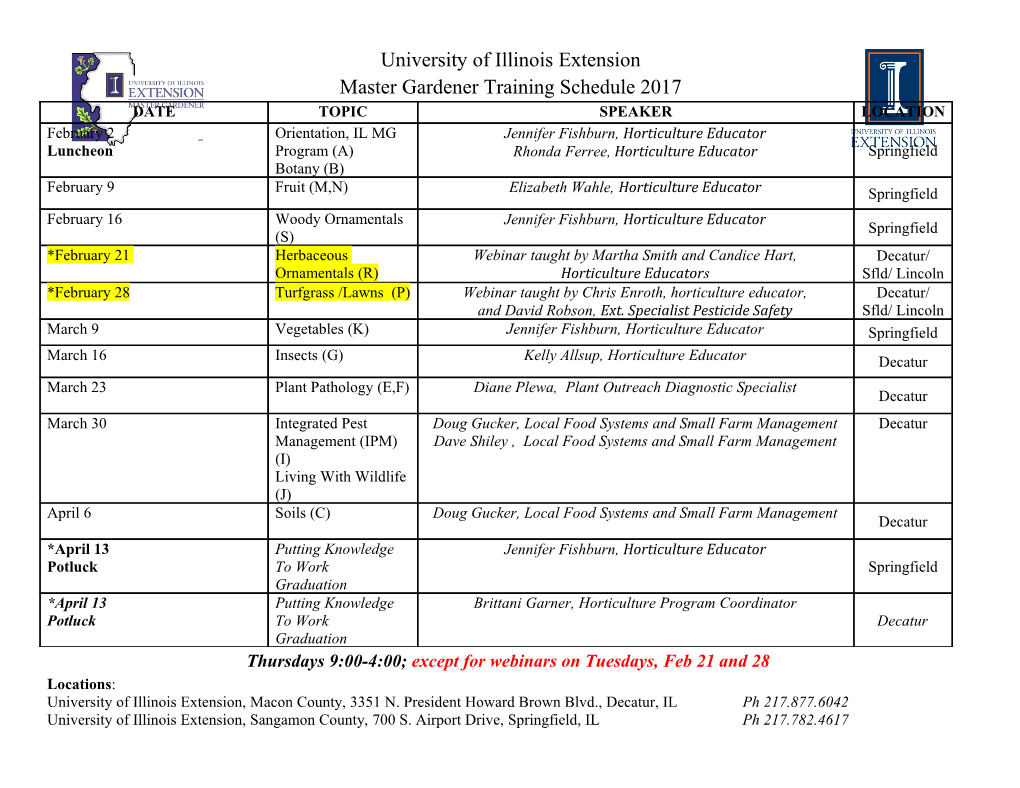
Ann Anat 186 (2004): 387–396 http://www.elsevier.de Bone strain gradients and optimization in vertebrate skulls Callum F. Ross1 and Keith A. Metzger2 1 Organismal Biology and Anatomy, University of Chicago, 1027East 57 St. Chicago IL 60637, USA 2 Anatomical Sciences, Stony Brook University, Academic Tower A, T-8, Stony Brook NY 11794-8081, USA Summary. It is often stated that the skull is optimally Introduction designed for resisting feeding forces, where optimality is defined as maximum strength with minimum material. Running counter to this hypothesis are bone strain gradi- In 1991, Hylander and colleagues reported that bone ents – variation in bone strain magnitudes across the skull strain magnitudes recorded from the supraorbital regions – which in the primate skull have been hypothesized to of macaques and baboons are much lower than those re- suggest that different parts of the skull are optimized for corded from the zygomatic arch, anterior root of the zy- different functions. In this paper strain gradients in the goma and mandibular corpus. They interpreted these data skulls of four genera of primates, Sus, and Alligator were to suggest that the supraorbital tori of macaques and ba- documented and compared. Strain gradients were perva- boons could be greatly reduced in size while still main- sive in all taxa sampled. Patterns of strain gradients taining an adequate safety factor to failure under routine showed inter-taxon differences, but strains in the mand- masticatory loads. They concluded (Hylander et al. 1991) ible and zygomatic arch were always higher than those in that their data provide “no good reason to believe that the circumorbital and neurocranial regions. Strain magni- enlarged browridges in living and/or fossil primates are tudes in Alligator were twice as high as those in mam- structural adaptations to counter powerful masticatory mals. Strain gradients were also positively allometric; i. e., forces”. Instead, they hypothesized that when the orbits larger primates show steeper gradients (larger differ- are positioned well rostral to the brain, they must be pro- ences) between the mandible and circumorbital region tected by sufficient bone to “prevent structural failure than smaller primates. Different strain magnitudes in dif- due to relatively infrequent non-masticatory external ferent areas of the same animal are hypothesized to re- forces associated with highly active primates (e. g., trau- flect optimization to different criteria. It is therefore matic accidental forces applied to the orbits and neuro- hardly surprising that the skull, in which numerous func- cranium)” (Hylander et al. 1991; see also Hylander and tional systems are found, exhibits very steep gradients. In- Ravosa 1992). ter-specific differences in strain magnitudes at similar In a later paper, Hylander and Johnson (1997) reported sites also suggest inter-specific differences in optimality that strain magnitudes also vary within the zygomatic criteria. The higher strain magnitudes in the Alligator arch, being higher anteriorly than posteriorly. They ar- skull suggest that the Alligator skull may be designed to gued that if optimality is defined as maximum strength experience extremely high strains less frequently whereas with minimum material, the extreme variability in bone the primate skull may be designed to resist lower strains strain magnitudes across the facial skeleton falsifies the more frequently. general assumption that the facial skeleton is optimized for resisting forces generated during routine mastication. Key words: Mastication – Feeding – Skull – Mandible – They reiterated the hypothesis that bone mass in excess Primates – Crocodilians – Mammals of that needed to optimize the facial skeleton to resist masticatory forces may make the facial bones “structu- Correspondence to: C. F. Ross rally adapted to counter infrequent nonmasticatory trau- E-mail: [email protected] matic loads” (Hylander and Johnson 1997). 0940-9602/04/186/05–06–387 $30.00/0 Subsequent work demonstrated that bone strain magni- variation in strain magnitudes – within the vertebrate tudes are also low in the supraorbital regions and inter- skull? Are strain gradients similar from one species to an- mediate in the lateral orbital walls of owl monkeys and other? What do strain gradients tell us about craniofacial galagos (Ross and Hylander 1996; Ravosa et al. 2000 a). Ravosa et al. (2000 a) reiterated the hypothesis that ex- cess bone mass in the facial skeleton (in this case, the lat- eral orbital wall) functioned “to ensure an adequate safety factor for accidental nonmasticatory forces”; they also suggested that there might be “positive allometry of the strain gradient” in the primate face, with larger ani- mals having lower strains in the supraorbital region be- cause of the positive allometry of circumorbital structures observed in morphometric analyses (Moss and Young 1960; Shea 1986; Ravosa 1988, 1991 a–c). Observations in owl monkeys, macaques and galagos of low strain magnitudes along the medial orbital walls, orbi- tal roofs and postorbital septa, thin plates of bone where traumatic blows were unlikely to fall, suggested to Ross (2001) that not all strain gradients reflect selection for in- creased resistance to traumatic blows to the head; he sug- gested a range of functions for thin plates of circumorbi- tal bone that experience very low strain magnitudes, including providing a rigid support for the brain and its meninges, deflecting chewing muscles around the orbital contents, and providing attachment for chewing muscles. Ross (2001) concluded that, “[a]ny role that these thin circumorbital bony plates perform in resisting feeding forces is coincidental and insignificant, and could be bet- ter performed by a more efficient distribution of bony tis- sue”. In sum, Hylander, Johnson, Ravosa and Ross have ar- gued that the primate facial skeleton is not optimally designed for resisting forces generated during feeding be- cause its various components perform a range of func- tions. Adaptations of the skeleton to these functions in- clude distributions of bone mass and bone shapes that are over-designed for resisting routine masticatory stresses: hence bone strain magnitudes recorded from these re- gions during routine mastication are low. These hypotheses regarding variations in strain magni- Fig. 1. Diagram of skull of Macaca illustrating gauge locations in tude (strain gradients) in primate skulls run counter to a primates and Sus. Abbreviations and region groups: Neurocra- long tradition in craniofacial biomechanics that the face is nium, pariet, parietal, Sus only; temp, adjacent to temporal line Macaca optimized for resisting masticatory loads (see references on frontal bone, only; Orbital region, dio, dorsal interor- bital; rio, rostral interorbital; dorb, dorsal orbital; roof, orbital in Hylander and Johnson 1997; Preuschoft et al. 1986 a,b). roof; bar, postorbital bar (anterior surface in Macaca, lateral sur- This tradition persists in recent presentations of finite-ele- face in Eulemur, Otolemur and Aotus); septum, postorbital sep- ment analyses of human skull morphology (Witzel and tum (intraorbital surface), morb, medial orbital wall; Zygomatic Preuschoft 1999, 2002); “Since we have found a far-reach- Arch, aarch, anterior 1/3 of zygomatic arch (Macaca only); ing correspondence between the spatial arrangement of march, middle 1/3 zygomatic arch, on zygomatic/jugal bone, im- stress-bearing regions and the bony structure of the skull, mediately anterior to zygomatico-temporal suture; parch, poster- the conclusion seems justified that the shape of the skull ior arch on zygomatic process of squamous part of temporal is essentially caused by biomechanic necessities. Charac- bone; zflg, zygomatic flange (Sus only); arz, anterior root of zy- teristic for all parts is a light-weight construction opti- goma, infraorbital of Hylander et al. 1991; Snout, max, maxilla; na, nasal (Sus only); pmax, premaxilla; Jaw Joint; qjug, quadrato- mised for minimal mass and maximal strength with the jugal; quad, quadrate; cond, surangular in Alligator, condylar best possible approach to minimal areas around given neck in Sus and Macaca; Mandible, symph, symphysis; avdent, volumes” (Witzel and Preuschoft 2002). anterior ventral dentary; pvdent, posterior ventral dentary; al- Published and new bone strain data relevant to these dent, anterior lateral dentary; pldent, posterior lateral dentary; hypotheses are presented here and the following ques- ang, angular; lingual, splenial in Alligator, lingual aspect of man- tions are addressed: How pervasive are strain gradients – dibular corpus in Macaca. 388 adaptation and evolution? To address these questions the Table 1. Mean and peak c-max data. available data on bone strain magnitudes in vertebrate Species Gauge location Mean Peak skulls are reviewed, characteristics of the strain gradients c-max (le) c-max (le) in different taxa are compared, and theoretical issues re- garding the meaning of these gradients are addressed. Eulemur dorsal interorbital 100 256 dorsal orbital 109 296 postorbital bar 284 676 zygomatic arch (middle) 530 1277 anterior root of zygoma 405 1420 Materials and methods mandibular corpus 599.5 Otolemur dorsal interorbital 334 1221 Sufficient data for an analysis of strain gradients are only avail- postorbital bar 398 1320 able for a limited number of species. The primate data are ana- medial orbital wall 346 609 lyzed at the generic level to increase the number of sites sampled mandibular
Details
-
File Typepdf
-
Upload Time-
-
Content LanguagesEnglish
-
Upload UserAnonymous/Not logged-in
-
File Pages10 Page
-
File Size-