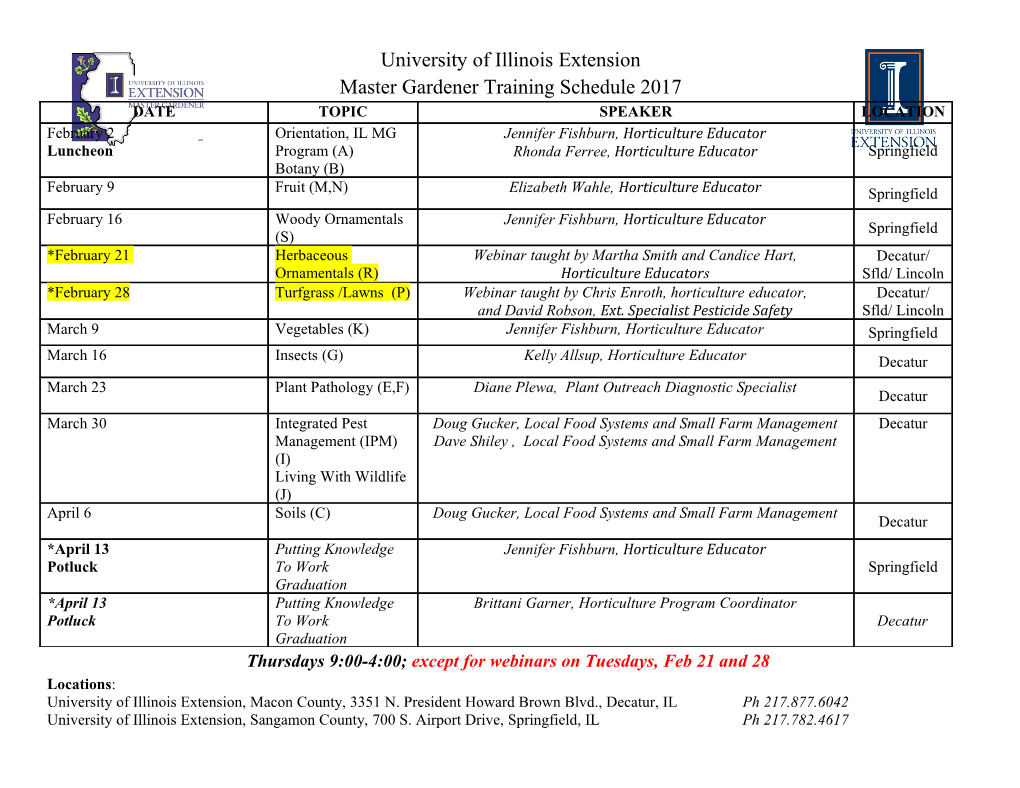
3.2 AN EVALUATION OF FDS AND CLARK COUPLED WILDFIRE MODEL Ruiyu Sun1 ∗ and Steven K. Krueger1 and Mary Ann Jenkins2 and William Mell3 and Joseph J. Charney4 1University of Utah, Salt Lake City, Utah 2York University, Toronto, Canada 3National Institute of Stardards and Technology,Gaiithersburg,MD 4United States Forest Service, North Central Research Station,Easy Lansing,MI 1. Introduction wildland fire, it is not possible to develop models that include all scales. Therefore, depending on the mod- Wildland fire is a natural phenomenon involving com- eler’s interests, each fire model concentrates on mod- plex chemical and physical processes. The range of eling wildfire behavior within a certain scale range. Ac- length scales for each process is large, from the sub- cording to Mell et al (2005), there are four basic types millimeter scale (combustion process) to the kilometer of models depending on whether the two interactions scale (convection process). In the evolution of a large are involved: operational models that do not explicitly wildland fire, the interactions between the flame and include the two interactions; models that mainly in- the fire plume and the local close–in and larger–scale volve the flame+plume/atmosphere interaction; mod- environment are important. The fires studied by Baum els that mainly involve the flame/fuel interaction; and (2000) can be characterized by the nature of their in- models that include both interactions to a greater or teraction with the local environment, where the local lesser degree. environment is defined by the geometry and burning The Clark coupled atmosphere–fire model (Clark et characteristics of the fuel bed, the properties of the am- al 1996; hereafter referred to as the Clark coupled bient close–in atmosphere, and the local topography. model) is an example of a wildfire model that empha- The two most important interactions therefore are be- sizes the interaction between the fire plume and the tween the fire flame and the fuel (hereafter referred atmosphere, and is designed to simulate wildland fires to as flame–fuel interaction), and between the fire over scales where a typical computational grid size of flame and plume and the ambient atmosphere (here- 10s of meters is too coarse to resolve physical pro- after referred to as flame+plume–atmosphere interac- cesses in the combustion zone. Evolving model winds tion). The flame–fuel interaction involves gas gener- from the lowest levels of the atmospheric fluid dynam- ation by solid fuel pyrolysis, the subsequent combus- ical model are passed to an operational empirically– tion of the fuel gases, and the resultant heat flux back based fire–spread–rate formulation (e.g., Hirsch 1996, to the solid fuel. The flame+plume–atmosphere in- Rothermel 1972) which is used to advance the fireline teraction involves the response of the fire flame and (Clark et al 2004). Because the thermal degradation of plume to the ambient atmospheric conditions and the the solid fuel is not modeled directly and combustion response of the atmosphere to the buoyant fire plume. is parameterized, computational resources are devoted This interaction can alter the orientation and geometry to resolving atmospheric physics. of the fire flame and plume, influencing the distribu- Examples of a wildfire modeling approach similar tion and intensity of the net heat flux to the solid fuel to the multiphase models that involve both inter- and the burning of the fuel (Mell et al 2005), and con- actions are the Fire Dynamic Simulator (FDS) and sequently changing the properties of local atmospheric FIRETEC. FIRETEC was designed at the Los Alamos conditions. National Laboratory (Linn 1997; Linn et al 2002; Linn It is extremely difficult to study wildland fires by di- et al 2005) and built to analyze complex wildfire behav- rect observation. Along with the cost and safety issues, ior that the current operational empirically–based wild- controlled fire experiments are practically impossible to fire models cannot represent. The FDS was designed at achieve in a natural setting. For these reasons current the Institute of Standards and Technology (NIST) and computational fluid dynamical models are important originally built to analyze industrial-scale fires. Mell et tools for the study of wildfire, especially severe wild- al (2005) has extended the FDS to simulate outdoor fire. fires in vegetative fuels (called WFDS for Wildland Fire Because of the wide range of scales involved in a Dynamics Simulator). In these models the pyrolysis of ∗ fuels and gas phase combustion are implemented in Corresponding author address: Department of Meteorology, University of Utah, Salt Lake City, UT 84112. E-mail: a numerical solution of the Navier-Stokes equations, [email protected] appropriate for low-speed, thermally-driven flow with an emphasis on smoke and heat transport from fires. was about 600 MW. Measurements were taken us- Computational resources are devoted to resolving fire ing a radiosonde-radiowind system, kite balloons, pho- combustion and the close–in fire/atmosphere flow over togrammetry from four observing stations, a ground the fire. network of temperature and wind velocity sensors, and Though capable of accounting for almost all the vari- an aircraft. The plume’s geometrical parameters, in- ables that exist in wildfire prediction, the computa- cluding radius (accuracy estimated at 10%) and verti- tional fluid dynamical models are not yet suitable for cal velocity at the visible boundary of the plume, delim- faster–than–real–time applications on today’s comput- ited by thick black smoke, were determined by a pho- ers. Such models can be used to improve our com- togrammetric method (Saporte 1966). Temperature prehension of wildfire and the accuracy of operational and vertical velocity measurements were made directly wildfire models to forecast actual fire spread and be- in the plume with a radiosonde system using a kite havior. Before applying these wildfire simulation mod- balloon. Below 600 m, plume–averaged vertical veloc- els to these problems, model validation is necessary. ity data were obtained with photogrammetry and the We need to compare model results to real fires. radio-sounding kite balloon, while at higher altitudes Proper model evaluation requires an appropriate set these data were obtained only with photogrammetry of field observations, taken under well–documented of the rising plume edge. and controlled conditions, and carefully analyzed for Benech (1976) claimed that the following plume fire behavior. In the so–called “Meteotron” fire experi- properties remained practically identical below the first ment, Benech (1976) presented the results of measure- 600 m of elevation for all experimental fires: ments in and around convective plumes initiated in the • atmosphere from the ground by an exceptionally pow- plume averaged radius at a given altitude; erful artificial heat source. The experimental project • plume vertical velocities at a given altitude; was designed to provide data for theoretical study of a 3 −1 stationary, non-spreading, convective fire plume. • vertical flux (m s ) at a given altitude, deduced The purpose of this report therefore is to use the from the former data; results from the Meteotron fire to examine how well • and temperature difference (hereafter referred to the FDS and Clark model depict low–level struc- as temperature excess) between the inside and the ture/properties of this type of fire plume. A fire outside of the plume at a given altitude. plume represents how the major portion of the en- ergy from the fire’s combustion process is put into the In the following sections, the FDS and Clark cou- atmosphere and the resulting buoyancy distribution. pled model simulations are compared to the Meteotron The plume is one mechanism for the flame+plume– mean plume radius, vertical velocity, mass flux, and atmosphere interaction. The turbulent characteristics temperature excess (temperature difference between of the plume also mean that surrounding air will be the plume–averaged temperature and environment– entrained into the plume and consequently a flow will averaged temperature) taken from Figures 5, 6, 7, and be induced in the air surrounding the fire. Once we 8 in Benech (1976), and the mean plume buoyancy flux demonstrate here how accurately the FDS and the constructed with these data. Clark coupled model simulate the properties and be- havior of a stationary, non-spreading plume, we can 3. Numerical Experimental Set-up begin to use WFDS, a physically–based multiphase fire model, to improve the fire parameterizations for A single FDS simulation and two Clark coupled model spreading fires utilized by wildfire coupled models and simulations were completed, and results from the sim- the forecast accuracy of operational models like FAR- ulations are compared to each other and to the obser- SITE. vational data from the Meteotron fire experiment. The version of FDS used in this study is described 2. The Meteotron Experiment by Mell et al (2005). The FDS domain was 400 m (x) × 400 m (y) × 600 m (z) and covered by a grid mesh From 1971 to 1973 Benech (1976) conducted eleven of 100 (x) × 100 (y) × 100 (z). The vertical grid was atmospheric fire plume experiments during the Me- stretched and vertical grid size was about 3 m near teotron fire experiment. The heat source consisted ground level. The model atmosphere was isothermal, of 97 burners arranged into a hexagonal area of with an ambient temperature of 30.85◦C. A uniform about 4000 m2 (radius 36 m). The experiments wind of 3 m s−1 and constant with height blew into generally lasted five to ten minutes, and in most of the domain from the west (in the positive x direction). the experiments the total theoretical thermal power To match the size and total heat release rate of the 2 Meteotron fires (4000 m2; 600 MW), a square fire of ing the plume soot density provided by the FDS simu- 63.25 m × 63.25 m was set.
Details
-
File Typepdf
-
Upload Time-
-
Content LanguagesEnglish
-
Upload UserAnonymous/Not logged-in
-
File Pages18 Page
-
File Size-