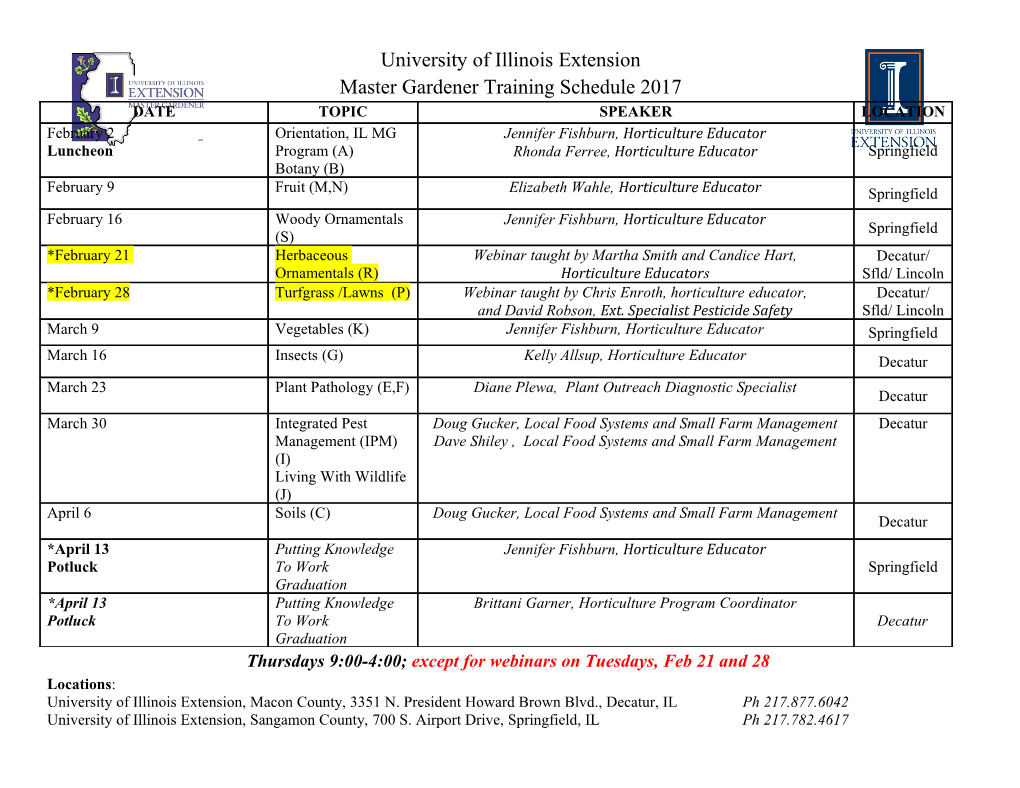
Coronal heating bservations of the solar corona go back 1: The inhomogeneous at least three millennia. One of the ear- and very structured Oliest records of the corona was made by solar corona (SOHO/EIT). Babylonian astronomers who reported during a solar eclipse in 1063 BC that “the day was turned to night, and fire in the midst of the heaven”. The discovery of spectroscopy in the late 19th century revealed the existence of a coronal “green line” at 5303 Å – a major puz- zle for astrophysicists for 50 years. The wave- length of this spectral line did not match any known elements and it was thought that a new element (coronium) had been discovered. The solution of this solar mystery came in 1939 when Edlén showed that the coronium line is emitted by highly ionized iron, Fe XIV, at tem- peratures well over 1 million K. Modern satel- lite observations from Skylab in the 70s, through SMM, Yohkoh and in present times SOHO, TRACE and RHESSI have revealed the solar atmosphere with unprecedented spatial and temporal resolutions covering wavelengths from (E)UV, through soft and hard X-ray to even γ-rays. Both spectroscopic and imaging instruments have contributed to the discovery of many and various fine-scale structures in the solar atmospheric zoo from small-scale X-ray bright points to large solar coronal loops (fig- ure 1). For an excellent textbook on the corona see Dolub and Pasachoff (1997). Once it was established in 1939 that the solar corona is much hotter than the photosphere, it Heating in the did not take long for the theoreticians to come up with reasonable mathematical models that tried to explain this apparently controversial fea- ture. The major problem is the observed behav- iour of temperature in the solar atmosphere: solar atmosphere energy is produced in the very hot (approxi- mately 14 million K) internal core part of the Robert Erdélyi reviews ways and means of heating the solar corona. Sun by thermonuclear fusion, then it propagates outwards, initially in the form of radiation (in Abstract small fraction of the total solar output (see table the radiation zone) up to about 0.72 R᭪ and 1). Estimates show that the total energy budget later by convection (in the convective zone) right The solar coronal plasma, mainly is just approximately 10–4 of the Sun’s total to the solar surface (photosphere), continuously confined in magnetic flux tubes, is energy output making it, in theory, relatively cooling the solar plasma. But after reaching its maintained at temperatures of several easy to suggest mechanisms to divert 0.01% of minimum at the top of the photosphere, the tem- millions of K. The heating process that the total solar output into heating the corona. perature starts to rise slowly throughout the generates and sustains the hot corona has chromosphere (up to around 20 000 K), fol- so far defied a quantitative understanding Solar magnetism lowed by a very steep increase in the narrow despite efforts spanning over half a As the spatial resolution of observations transition region (a few 100 000 K) up to century. In this paper I review the most improved, more and more structures were dis- around 2 million K in the corona (figure 2). If popular and viable mechanisms of heating covered at the solar surface and in the solar one goes continuously away from the source of the solar atmosphere, from low atmosphere. Examples of well-known large- the energy, the solar core, one would expect a chromospheric levels through the scale structures are sunspots, complex active monotonic temperature decrease; this tendency transition region up to the corona. I regions, prominences, coronal loops and coro- is reversed above the temperature minimum and address two principal questions: What is nal holes; while examples of fine structures are the temperature actually increases in the solar the source of plasma heating in the solar the magnetic pores, dark mottles, spicules, atmosphere (figure 2). (and stellar) atmosphere? How do supergranular cells, filaments, X-ray and EUV The existence of the corona requires an input perturbations dissipate efficiently, bright points. One of the earliest discoveries in of energy above that which would occur by resulting in hot plasmas? The latest solar physics was the solar cycle, i.e. Hale’s thermodynamic relaxation from the photo- results of theoretical and observational polarity law, the butterfly diagram for sunspots, spheric output. The excess of this non-thermal studies provide some answers, but there the cyclic variations in sunspot numbers (dis- (sometimes also called mechanical) energy to remains much to be learned. cussed in more detail in this issue by Tobias and sustain the solar corona is surprisingly just a Weiss on pages 4.28–4.33, and Bushby and 4.34 August 2004 Vol 45 Coronal heating TR photosphere chromosphere corona 1024 106 Mg X (625 Å) N Ne VIII (770 Å) ) 1022 –3 O VI (1038 Å) ity N (mity N re T (K) O IV (790 Å) s u 5 10 C IV (1548 Å) 20 en 10 d Si IV (1394 Å) temperat C II (1037 Å) particle He I (584 Å) 1018 Lyα (1215 Å) 104 1016 T 3: The solar corona in the 171 Å SOHO EIT spectral line (upper left) and the corresponding SOHO MDI magnetogram 100 1000 10000 (lower right) at photospheric levels. Magnetic τ altitude above 5000 = 1 (km) field concentrations coincide with bright patches in the SOHO EIT image, indicating the 2: Temperature (red line) and density (yellow line) distributions as a function of the height measure in role of magnetic field in the process of km in the solar atmosphere. The formation of popular lines for observations is indicated by green. coronal heating. Note the logarithmic scales. (Courtesy H Peter.) 8–20×106 K, while in open mag- of a specific favoured heating mechanism Table 1: Average coronal energy netic regions such as coronal (Cargill 1993) and confirm these signatures by –2 –1 holes, maximum temperatures observations (e.g. generated flows, specific spec- losses (in erg cm s ) may only be around 1–1.5× tral line profiles or line broadenings, etc). 106 K. Next, observations also The heating process comprises three phases: Loss mechanism quiet Sun active region coronal hole show that temperature, density the generation of a carrier of energy; the trans- Conductive flux 2×105 105–107 6×104 Radiative flux 105 5×106 104 and magnetic field are highly port of energy into the solar atmosphere; and, Solar wind flux <5×104 <105 7×105 inhomogeneous. Fine structures finally, the dissipation of this energy in the var- Total flux 3×105 107 8×105 (e.g. filaments in loops) may ious structures of the atmosphere. Usually it is have 3–5 times higher densities not difficult to establish a theory that drives an than is usual in their environ- energy carrier without contradicting observa- Mason on pages 4.7–4.13). It soon turned out ment. An interesting constraint is the contrast tions. Neither does the literature seem to be that these temporal phenomena are linked to between fluctuating brightness and the associ- short of transport mechanisms. There is, how- the internal generation mechanism of the global ated fluctuating velocities and the quasi-static ever, real hardship in understanding how the solar magnetic field. Skylab observations made nature of the corona. There is little known transported energy is dissipated efficiently. A it clear that there is a strong correlation about how the heating depends on magnetic summary of the heating mechanisms is given in between X-ray emitting hot and bright coronal field strength, structure size (length, radius, table 2 (Ulmschneider 1998). regions and the underlying surface magnetic expansion) and age. The heating mechanisms that operate in the field concentrations suggesting that coronal We are mainly interested in solar (and stellar) solar atmosphere can be classified depending on heating and solar magnetism are intimately atmospheric heating mechanisms that can pro- whether they involve magnetism or not (Narain related (figure 3). vide a steady supply of energy to balance the and Ulmschneider 1996). If the heating mecha- Similar correlations were found between the atmospheric (chromospheric and coronal) nism operates in magnetism-free regions (e.g. in ubiquity of small-scale structures and the mag- energy losses, although this energy does not need the chromosphere of the quiet Sun) one can netic solar cycle. Very recent studies of the to be produced in a steady way, i.e. random work within the framework of hydrodynamics photospheric magnetic carpet also seem to sug- energy releases that produce a statistically aver- and such heating theories can be classified as gest that such correlations exist even at small- aged steady state are allowed for. Observational hydrodynamic heating. Examples of such heat- est scales (discussed by Proctor on pages tests of a specific heating mechanism may be dif- ing mechanisms could be acoustic waves and 4.14–4.20 of this issue). ficult because several mechanisms might operate pulsations. On the other hand if the plasma is at the same time. Theoretical estimates often embedded in magnetic fields the framework of Observational constraints predict very small spatial scales where the ulti- magnetohydrodynamics (MHD) may be the An acceptable model of solar (and stellar) mate dissipation occurs, sometimes of the order appropriate approach and these theories are atmospheric heating has to comply with a few of a few hundred metres, that even with current called MHD heating mechanisms (Browning observational facts (Cargill 1993, Zirker 1993). high spatial resolution satellite techniques can- 1991, Gomez 1990, Hollweg 1991, Pries and It is now evident the solar atmosphere is highly not be resolved – and will not be for a while.
Details
-
File Typepdf
-
Upload Time-
-
Content LanguagesEnglish
-
Upload UserAnonymous/Not logged-in
-
File Pages4 Page
-
File Size-