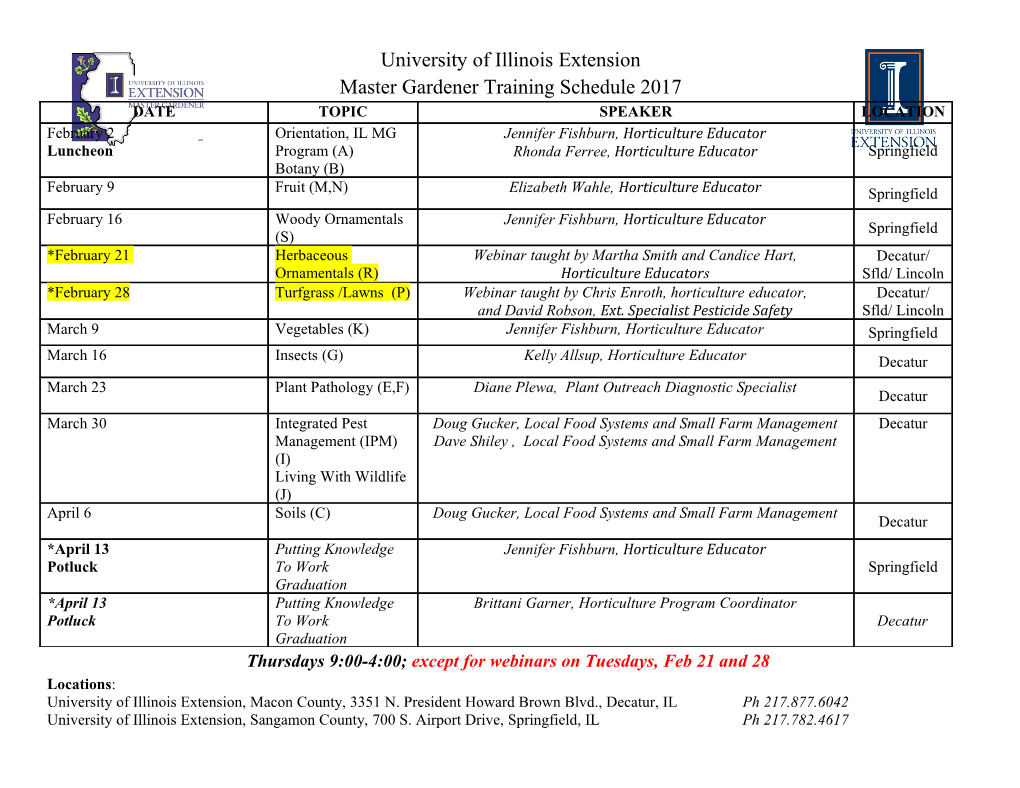
In Proceedings of the 9th ESA Workshop on Advanced Space Technologies for Robotics and Automation 'ASTRA 2006' ESTEC, Noordwijk, The Netherlands, November 28-30, 2006 DEMONSTRATING REAL-WORLD COOPERATIVE SYSTEMS USING AEROBOTS Ehsan Honary1, Frank McQuade1, Roger Ward1, Ian Woodrow2, Andy Shaw3, Dave Barnes3, Matthew Fyfe4 1SciSys [email protected], [email protected], [email protected] Clothier Road, Bristol BS4 5SS, UK TEL: +44 (117) 9717251, FAX: +44 (117) 9721846 2SEA [email protected] Systems Engineering & Assessment Ltd, Beckington Castle, Castle Corner, Beckington, Frome BA11 6TB, UK, TEL: +44 (1373) 852120, FAX: +44 (1373) 831133 3University of Wales Aberystwyth Andy Shaw: [email protected], Dave Barnes: [email protected] Computer Science Department, Penglais, Aberystwyth, Ceredigion, SY23 3DB, Wales, UK TEL: +44 (1970) 621561, FAX: +44 (1970) 622455 4SCS [email protected] Systems Consultants Services Limited, Henley-on-Thames, Oxfordshire, England, RG9 2JN TEL: +44 (1491) 412102, FAX: +44 (1491) 412082 ABSTRACT Use of multiple autonomous robots for practical applications has become more important over the years [1][2][3][4]. The potential for applications of collective robotics is high, in particular in the aerospace environment. SciSys has been involved in the development of Planetary Aerobots funded by ESA for use on Mars and has developed image-based localisation technology as part of the activity. It is however possible to use the Aerobots in a different environment to investigate issues in regard with robotics behaviour such as data handling, communications, limited processing power, limited sensors, GNC, etc. This paper summarises the activity where Aerobot platform was used to investigate the use of multiple autonomous Unmanned Underwater Vehicles (UUVs), basically simulating their movement and behaviour. The main purpose of the Multiple UUV Operations (MuOps) project within the BAUUV1 program was to identify and de- risk critical UUV components and raise the technology readiness level of the components required for the operation of multiple cooperative vehicles. This paper reports the computer simulations and the real-world tests and the lessons learnt from them. 1. INTRODUCTION Autonomous underwater operations are an attractive solution for challenges confronted in modern battlefields. A swarm of UUVs can collectively co-operate together to carry out missions or to survey a targeted area. Robots operating in land, underwater, air or space can all benefit from collective activity and despite the differences between the environments, the same principles and solutions can be applied to all of them. 1 The BAUUV program (Battlefield Access Unmanned Underwater Vehicle) was led by SEA on behalf of UK MOD. SciSys Ltd. led the consortium to complete the MuOps study with contributions from University of Wales, Aberystwyth (UWA) and SCS. The MuOps study consisted of three main phases: 1. Operational concepts. A series of scenarios were considered as part of the MuOps study. Scenarios such as Anti- submarine warfare, Littoral Beach Operations, Harbour Operations, Surveillance, etc. were considered and based on a trade off table eventually Mine Counter Measures (MCM) was selected as the most appropriate scenario to test the multi-UUV principle. 2. Simulation of underwater vehicles. This included 5 degrees of freedom, ocean model, buoyancy, inertia matrices to calculate motion underwater, drag and PID routines to control the robot. A behaviour-based approach was used in conjunction with minimal collective robotics principles to guide a swarm of robots underwater. The scenarios consisted of MCM, were the swarm was responsible to detect, classify and dispose of mines in the target area. 3. Demonstration with physical hardware. The algorithms were subsequently put onboard Aerobots (Autonomous flying balloons) to validate the simulation results with real-world experiments. Aerobots were used as an analogy to UUVs to move freely in a 3D space. The Aerobots were tracked with a positioning system and horizontal and vertical thrusters were used to navigate the robots around the lab environment. The focus of this paper is on the implementation of the command and control algorithms and their deployment onto the Aerobot platform. 2. SIMULATION ARCHITECTURE The simulation tool is divided into four primary blocks with their corresponding sublevel modules as shown in Fig 1 (Left). • Dynamics Module. Dynamics of all UUV’s and the underwater environment simulation: o Environment Model. o Translational Dynamics o Attitude Dynamics • Guidance and Sensors. This module performed Guidance, Navigation and Control management: o Sensors and Navigation o Behaviour. Controlling the overall behaviour of a UUV o Guidance, control (PID), and propulsions models. 3. GUIDANCE ARCHITECTURE The purpose of the guidance algorithms is to generate the target Cartesian coordinates for the location of the vehicle in the next time step. The control module (either in the simulation or in the real world) is then responsible for implementing the described commands. By continuously generating a series of target points the UUVs can be controlled to move and function within the environment. In simulations, the calculation of the eventual behaviour of UUVs is carried out in the guidance algorithms. This means that commands for the actuators (such as ‘fire weapon’, or ‘communicate’) are all issued within this block. Special care has been taken into account to create an architecture which allows the generation of multiple scenarios, based on different number of UUVs and different behaviours that can be active at any one time. The architecture of the guidance algorithms was based around the implementation and use of multiple behaviours for different purposes. The core of the architecture was designed using the DAMN (Distributed Architecture for Mobile Navigation)[5]. The architecture is shown in Fig 1 (Right). 2 Mode Manager weights votes Maximum depth Mine classification & control disposal Mine detection Arbiter Supervisor duties Trajectory following Flocking Minimum altitude votes control Other behaviours … Commands UUV Controller Fig 1. (Left) Simulations modules and their interconnection. (Right) Guidance architecture. Groups of distributed behaviours communicate with a centralised command arbiter. Each behaviour is responsible to control certain aspects of the UUVs functionality. The behaviour sends its output to the arbiter. These outputs tend to satisfy the behaviour’s objectives. The arbiter is then responsible to combine the outputs from various behaviours and generate actions based on that. Multiple outputs are combined by weighting. A high level mode selection system controls the overall operation of the UUVs. 4. INFLUENTIAL PARAMETERS In the context of MCM, there are a variety of scenarios that can take place. Certain parameters have more impact on the overall performance than others. The following are perhaps the most important influential parameters in the context of multiple UUV operations: • Cooperation versus non-cooperation. • Heterogeneous swarms versus homogenous swarms. • Variations on number of UUVs. • Cooperation using various algorithms such as flocking, formation flying and leader-follower. • Impact of communication (or lack of it) on cooperative solutions. • Analysis of coverage, detection, classification and disposal rates of mines under different scenarios. 5. THE MCM SCENARIOS The intention in this study was to shed light on these issues and to provide results based on the simulation and then analyse and justify the conclusions. For this, two sets of scenarios were selected: • Scenario 1: Detection (Coverage). This contained the following cases: Single UUV, Non-cooperative UUVs and Cooperative UUV swarm. • Scenario 2: Detection, classification and disposal. This contained the following cases: Single disposal UUV, Homogenous disposal swarm (all members identical), Heterogeneous disposal swarm (with different types of robots) 3 6. UUV TYPES This section briefly summarises the 3 main types of UUVs used in simulations and demonstrations. The actual requirements for each UUV depend on the selected scenario and solution. • Type I: Detection UUV. This UUV is equipped with appropriate sensors (such as sonar) for mine detection purposes. • Type II: Communication UUV. This is a communication UUV that is capable of communicating with the outside world as well as with other UUVs. Hence, it can be the point of contact for a group of UUVs. • Type III: Classification & Disposal UUV. This UUV is capable of classifying a detected mine-like object. Once it has classified the mine it can move on to dispose it by using its finite number of ‘disarming’ weapons. This UUV need to be rearmed every time the weapons are depleted, by returning to a predefined replenishing point. 7. SIMULATION OF SCENARIOS As part of the simulation phase, scenario cases were simulated case by case. Key parameters (such as drift, number of UUVs, communications, etc.) were varied for multiple runs and the results were graphed and analysed. Measures of performance such as coverage and time to carry out the mission were used to compare the results between the cases. The UUVs and ocean environment were simulated in Matlab-Simulink. The results were later visualised in our in-house 3D visualisation program. As part of this exercise different cooperative algorithms such as hybrid flocking, leader follower and formation flying were considered. Continuous and periodical
Details
-
File Typepdf
-
Upload Time-
-
Content LanguagesEnglish
-
Upload UserAnonymous/Not logged-in
-
File Pages8 Page
-
File Size-