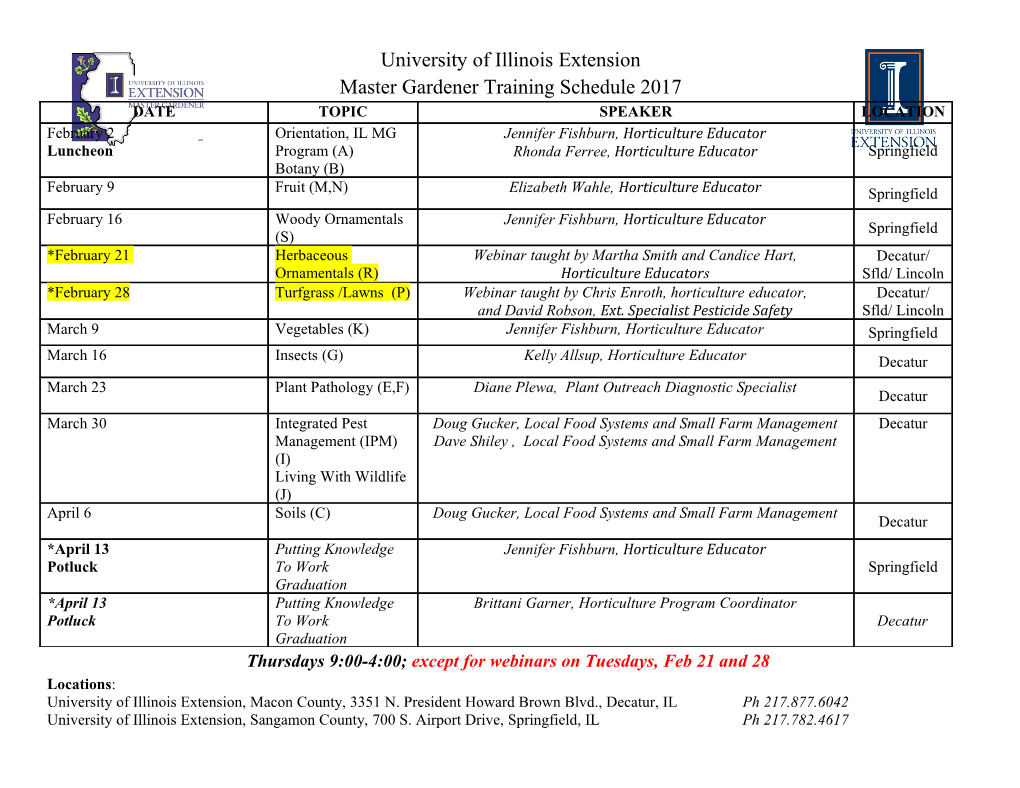
Deep Mining 2017: Eighth International Conference on Deep and High Stress Mining – J Wesseloo (ed.) © 2017 Australian Centre for Geomechanics, Perth, ISBN 978-0-9924810-6-3 doi:10.36487/ACG_rep/1704_07_Dineva Evolution of seismicity at Kiruna Mine S Dineva Luleå University of Technology, Sweden M Boskovic Luossavaara-Kiirunavaara Aktiebolag (LKAB), Sweden Abstract Kiirunavaara (Kiruna) iron ore mine owned by LKAB (Sweden) is one of the largest underground mines. Mining started in 1898 as an open pit mine. In mid-1950, the mine started a transition to underground mining and passed to only underground mining in 1962. More substantial problems with seismicity started in 2007-2008 when the deepest mining level was 907 m (ca. 670 m below surface). By 2016, the mining production is at 1,022–1,079 m Level (ca. 785–845 m below surface). More than one billion tonnes of ore have been extracted since the beginning of mining. The average yearly production in recent years is 28 million tonnes. By 2016 the mine has the largest underground seismic system in the world with 204 operational geophones. The number of the sensors (geophones with natural frequencies of 4.5, 14, and a few of 30 Hz) changed with the increasing of production depth. The major stages with seismic system upgrades are: August 2008–June 2009 with 112 installed geophones, and July 2012–September 2013 with 95 installed geophones. During 2016–2017 it is planned to install some additional 45 geophones. The study was carried out to identify some trends in seismicity as the mining goes deeper and to find the correlation with some main controlling parameters – volume and depth of the production in order to obtain information for future seismic hazard and risk analysis. Custom made applications within mXrap were utilised to carry out the spatial variations of seismicity. The analysis showed substantial difference between the seismicity in the three studied blocks – 15/16, 28/30, and 33-37/34, with the weakest seismic activity in Block 15/16 (Mmax 1.6, maximum observed magnitude), followed by Block 28/30 (Mmax 2.2), and then largest seismicity in Block 33-37/34 (Mmax 2.2). The daily seismicity rate increased substantially through the years only for Block 33-37/34. The seismicity correlates strongly with the production depth. In general a straightforward correlation between the production volume and number of larger events (M > 0) was not found for the three studied blocks, assuming there are other factors affecting the seismicity, e.g. geological structures, areas with contrast in geomechanical properties, etc. The spatial variations of some seismic source parameters were traced for varying periods of time, depending on the major production stages (opening of new levels, full production, closing) for the three blocks. The distributions of the cumulative seismic energy showed a maximum around and below the production. The cumulative seismic moment and number of events in most cases showed a maximum around and above the production, indicating caving in these areas. The static stress drop shows the largest values around and below the production on the footwall side, corresponding also to the areas with increased stress. The energy index showed increased stresses in the same areas (EI > 1). This study is only the first overview of the seismicity in Kiruna Mine. For seismic hazard assessment and risk analysis further more detailed studies with smaller time intervals need to be carried out to obtain more precise correlations between the seismic parameters and the production volume and depth, and other possible factors affecting seismicity (geological structures, areas with contrast geomechanical properties, etc.). Keywords: mining-induced seismicity, underground deep mining, rockbursts 1 Introduction Kiirunavaara (Kiruna) iron ore mine owned by LKAB (Sweden) is one of the world’s largest underground mines. Mining started in 1898 as an open pit mine. In mid-1950, the mine started a transition to underground mining Deep Mining 2017, Perth, Australia 125 Evolution of seismicity at Kiruna Mine S Dineva and M Boskovic and passed to only underground mining in 1962. More substantial problems with seismicity started in 2007–2008 when largest mining depth was at Level 907 (670 m below the surface). By 2016, the mining production is at Levels 1,022–1,079 (785-855 m below the surface). More than one billion tonnes of ore have been extracted since the beginning of mining. The average yearly production in recent years is 28 million tonnes. By 2016 the mine has the largest underground seismic system with 204 operational geophones. The number of the sensors (geophones with natural frequencies of 4.5, 14, and a few of 30 Hz) changed with the increasing of production depth. The major stages with seismic system upgrades are: August 2008–June 2009 with 112 installed geophones and July 2012–September 2013 with 95 installed geophones. During 2016–2017 it is planned to install an additional 45 geophones. The mining method in Kiruna Mine today is sublevel caving where crosscuts are driven from the footwall through the orebody with a distance of 25 m between them. The spacing between production levels is 29 m. Fan shaped patterns with 10 to 13 holes and 3 m burden are drilled with remote controlled rigs in all crosscuts. The fans are charged with approximately 2,500 kg of emulsion and each fan contains around 10,000 t of ore. Electric load–haul–dump machines remove the ore to orepasses that connect to the main haulage level at 1,365 m. The ore is loaded by gravity from the orepasses into remotely controlled shuttle trains that transport the ore to a series of primary crushers. The ore is then hoisted in two stages to the sorting plant at the surface. In Kiruna Mine the orebody is divided into 10 production blocks, named according to the north–south direction (Y coordinate). During the transition from old haulage level at 1,045 to the new one at 1,365 Level, the names and borders between production blocks have been changed and the new production blocks named according to the new Y coordinates down to the 1,400 m Level (Z). In the east–west direction (X) the borders of the studied blocks are defined by the distance of the infrastructure in the footwall (100 m from the footwall structure) and hanging wall contact (defined by the orebody in mXrap). Here the seismicity was studied in three blocks, which are defined by the new coordinates from the surface down to 1,400 m Level. The coordinate ranges of the studied blocks are shown in Table 1. Table 1 Coordinate ranges for the studied Block 15/16, Block 28/30 and Block 33-37/34 Block East–west (X) North–south (Y) Up–down (Z) Start End Start End Start End B15/16 6,000 6,500 1,400 1,800 -236 -1,400 B28/30 6,000 6,600 2,720 3,100 -200 -1,400 B33-37/34 6,100 6,700 3,100 3,700 -200 -1,400 The aim of this study was to follow the developments in seismicity with the mining going deeper starting from the end of 2008, when significant induced seismicity started, until July 2016: We wanted to see if the number of events and maximum observed magnitudes increased during the study period. We followed the mining seismicity in depth to find if it correlates with the production depth and production volume. We explored the spatial variations in seismic source parameters (cumulative released seismic energy and seismic moment (co-seismic deformation)) during the production phases and possible correlation between the two parameters. We looked at spatial variations of some stress indicators (average stress drop and energy index) during the production phases. 126 Deep Mining 2017, Perth, Australia Seismic monitoring and analysis The analysis was done block-by-block and level-by-level (for the production phases) and the results were compared. The final aim was to gain insights into the seismic process as a function of some main controlling factors, production volume and depth that could be used later for seismic hazard and risk assessments, as well as to find possible indicators of increased seismicity or larger seismic events caused by the larger depth of mining. The most important tool used in this study was the mXrap software (Harris & Wesseloo 2015), employing the Grid Based Analysis app (Wesseloo & Harris 2015) explained in more detail in Wesseloo et al. (2014). This mXrap app was custom modified by Johan Wesseloo and Paul Harris (Australian Centre for Geomechanics) with new applications for the purpose of this study. As Kiruna Mine is almost 4,500 m long and the seismicity rate is high we concentrated only on a few areas (blocks) with different characteristics of seismicity and frequency of rockbursts – Block 15/16 (with comparatively low seismicity), 28/30 and 33-37/34 (with highest seismicity and number of rockbursts). The seismicity for a one-year period (June 2015–July 2016), the position of the studied blocks, and the seismic sensor locations by 2016 are shown in Figure 1. Figure 1 (a) Seismicity in Kiruna Mine for period June 2015–July 2016; (b) outline of studied blocks; (c) seismic sensor locations by 2016. The size of the spheres representing the seismic events (a) is proportional to the local magnitude M. The orebody is shown as a shaded area. The production Deep Mining 2017, Perth, Australia 127 Evolution of seismicity at Kiruna Mine S Dineva and M Boskovic levels between 2008 and 2016, as well as the orepasses and the haulage level are also shown. The isolines on the top of each plot are the surface topography In order to discriminate orepass noise (recorded non-seismic events, as dumped rock bouncing in the orepass), an ellipsoidal volume with a horizontal section of 50 × 3 m along the orepasses was excluded from the analyses.
Details
-
File Typepdf
-
Upload Time-
-
Content LanguagesEnglish
-
Upload UserAnonymous/Not logged-in
-
File Pages17 Page
-
File Size-