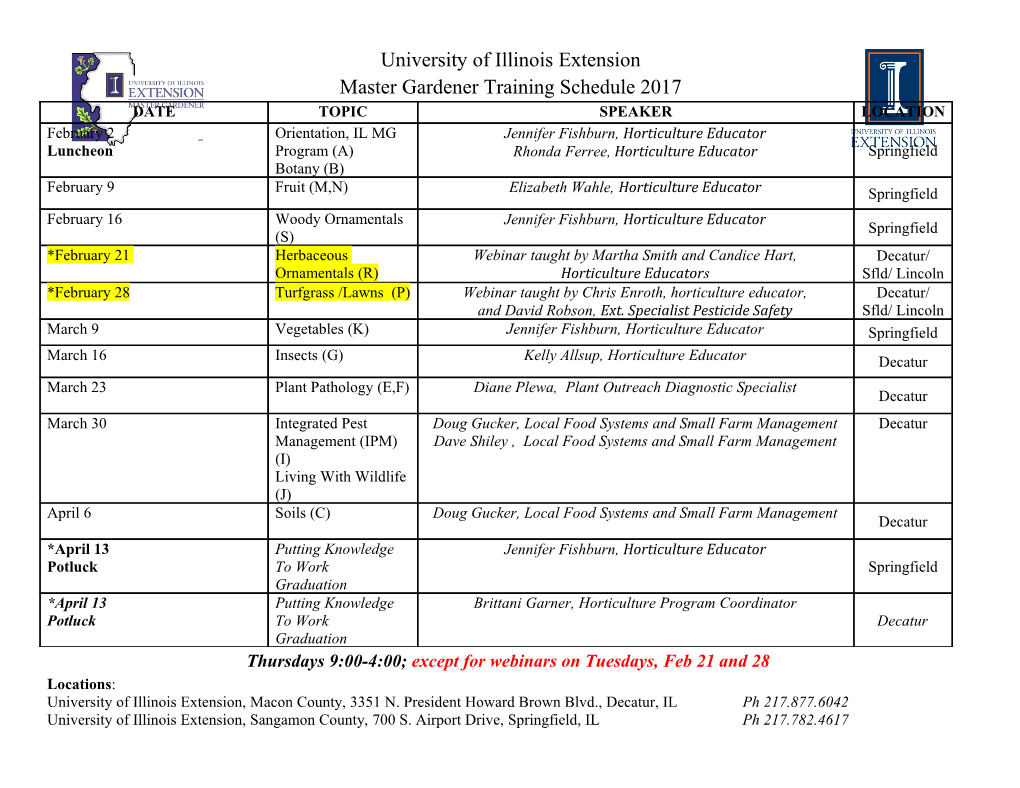
University of California Los Angeles On the thermodynamic efficiency of a multiferroic thermomagnetic generator: From bulk to atomic scale A dissertation submitted in partial satisfaction of the requirements for the degree Doctor of Philosophy in Mechanical Engineering by Samuel Mancilla Sandoval 2014 c Copyright by Samuel Mancilla Sandoval 2014 Abstract of the Dissertation On the thermodynamic efficiency of a multiferroic thermomagnetic generator: From bulk to atomic scale by Samuel Mancilla Sandoval Doctor of Philosophy in Mechanical Engineering University of California, Los Angeles, 2014 Professor Gregory P. Carman, Chair A unique multiferroic type of thermomagnetic generator is being investigated in order to establish its thermodynamic efficiency at different size scales. This device generates electricity when a magnetic material interacts with a thermal gradient by means of a spring-magnet mechanism. This unique technology is compared to other thermal-electric energy harvesting technologies to show that these devices have a similar goal of achieving a maximum theoretical efficiency of around 50% relative to Carnot. The first approach towards achieving improved performance relies on the analytical modeling, and experimental verification, of several sub- systems stemming from the original design, which include the optimization of the magnetic force component, the optimization of the heat transfer process and the efficiency of the energy conversion process. The method to improve the magnetic force component is not recommended and neither is the method to improve the heat transfer process. Nevertheless, the energy conversion subsystem is success- fully modeled and verified; thereby suggesting that an electromagnetic induction coil may be better suited for the energy conversion process over a ferroelectric transduction mechanism at bulk scale. A cascade design is also investigated as a method to improve device efficiency; though analysis reveals a design flaw, which ii leads to other methods for improving efficiency. Two models of thermomagnetic generator thermodynamic efficiency are devel- oped, which are based on distinct approaches taken by Solomon and Brillouin in order to compare this unique system to a Carnot engine. The model based on a modified form of Solomon's approach results in a relative efficiency of 0.5%, which compares well with an estimate of efficiency based on provided data from the orig- inal design. This representative model of efficiency was then applied to a survey of pure elements for comparison, which confirms gadolinium as the best material for use as a working body with a relative efficiency of around 20% . The model based on Brillouin's approach represents a more rigorous thermodynamic analysis, which qualitatively agrees with the results based on Solomon's approach, though it predicts larger values of efficiency for most of the materials in the survey. The conservative model based on Solomon's approach is then applied to a hypothetical system that uses a single-domain magnetic material as a working body. This effort is pursued since single-domain nanostructures exhibit a remanent magnetization, which is shown to increase magnetic energy density. The resulting analysis pre- dicts efficiencies on the order of 30% relative to Carnot for this nanoscale system, though the effects of size are not considered in this model. A model is developed to correlate the effects of size on thermodynamic effi- ciency for this device. Considering a nanoscaled nickel structure as a working body, this model combines three existing models to predict relative efficiency values that are comparable to the bulk scale, although this system may operate closer to room-temperature. This result is unexpected since the absolute efficiency is shown to increase as a function of decreasing size, though this discrepancy is explained as a consequence of Curie point suppression. The combined model is also applied to a hypothetical composite made of separated layers of nickel with distinct thicknesses. This composite material is predicted to spread the ferro- magnetic transition across a much larger temperature range as compared to bulk iii nickel, such that this material may be better suited for different applications; for example, as a sensor or as a thermal switch. Moreover, this combined model is also shown to be a lower-bound estimate of thermodynamic efficiency, since the actual performance depends on material characterizations that have yet to be determined. The magnetization of ferromagnetic nanostructures as a function of both ap- plied field, and temperature represents a current engineering challenge. This may be due to current manufacturing techniques that produce defects, and may also be due to resolution limitations of commercially available magnetometers. These challenges also affect a proposed hypothesis regarding the existence of a distri- bution of transition temperatures within a single nanostructure. This hypothesis aims to resolve whether the diffuse transition behavior of ferromagnetic nanostruc- tures is due to volume-averaged magnetometer measurement techniques, and/or due to surface effects from defects resulting from imperfect manufacturing tech- niques. These issues would need to be resolved before considering a nanoscale design, which may potentially be useful as a secondary energy recycling device in thermally-assisted magnetic recording applications. Nevertheless, a proof-of- concept experimental setup is offered that may be useful for future designs at the smallest relevant scales. iv The dissertation of Samuel Mancilla Sandoval is approved. Chistopher S. Lynch Adrienne S. Lavine Bruce S. Dunn Gregory P. Carman, Committee Chair University of California, Los Angeles 2014 v Dedico esta tesis a mis maravillosos padres Marcia y Narciso, mi bella esposa Dayna y nuestra querida Foxy, mis incre´ıbleshermanos Sandra, Monica y Ernesto, y a todos mis amigos. vi Table of Contents 1 Introduction :::::::::::::::::::::::::::::::: 1 2 Thermal-Electric Energy Harvesting Review ::::::::::: 6 2.1 Introduction . .6 2.2 Thermoelectrics based on the Seebeck effect . .7 2.3 Thermomagnetic Generators . 10 2.3.1 Ferromagnetic Phase Transition . 12 2.3.2 Energy Harvesting based on Electromagnetic Induction . 19 2.3.3 Energy Harvesting based on Multiferroics . 20 2.4 Pyroelectric Generators . 22 2.5 Summary . 23 3 Experimental Approach to Improve Performance :::::::: 24 3.1 Introduction . 24 3.2 Magnetic Energy . 25 3.3 Heat Transfer . 29 3.4 Harvest by Electromagnetic Induction . 33 3.4.1 Analytical Modeling . 34 3.4.2 Experimental Test Setup & Results . 37 3.4.3 Other Considerations . 42 3.5 Summary . 45 4 Thermodynamic Efficiency Analysis of a Multiferroic Thermo- magnetic Generator ::::::::::::::::::::::::::::: 46 vii 4.1 Introduction . 46 4.2 Solomon Efficiency . 47 4.2.1 Process 1 − 2: Heat rejection at constant field . 50 4.2.2 Process 2 − 3: Applied field at constant temperature . 51 4.2.3 Process 3 − 4: Heat input at constant field . 53 4.2.4 Process 4 − 1: Spring restoration at constant temperature 53 4.2.5 Cycle Analysis . 54 4.3 Brillouin efficiency . 59 4.4 Magnetic Single Domain Considerations . 63 4.5 Summary . 66 4.6 Appendix . 67 5 Size Effects on Efficiency :::::::::::::::::::::::: 75 5.1 Introduction . 75 5.2 Background . 76 5.2.1 Model 1 - Thermodynamic Efficiency . 76 5.2.2 Model 2 - Ferromagnetic Transition . 77 5.2.3 Model 3 - BOLS Correlation . 80 5.3 Combined Model . 85 5.4 Results & Discussion . 87 5.5 Summary . 93 6 Other Considerations :::::::::::::::::::::::::: 94 6.1 Introduction . 94 6.2 Transition Temperature Distribution . 94 viii 6.3 Design of a NanoMTMG . 100 6.4 Summary . 102 7 Conclusion ::::::::::::::::::::::::::::::::: 103 References ::::::::::::::::::::::::::::::::::: 106 ix List of Figures 1.1 Multiferroic thermomagnetic generator (MTMG) with restoring spring mechanism (Images borrowed from Ujihara et al., 2007 [UCL07]); (a) Actual device, (b) cross-section during cooling, (c) cross-section during heating. .2 1.2 Illustration of a ATP synthase (Image borrowed from Stock et al., 1999 [SLW99]) to harvest energy (left) compared with our fun- damental study element (Images borrowed from T. Chung et al., 2009 [CKC09]) Ni nanobar (i.e. 150nm x 30 nm x 50nm) as a po- tential working body in thermomagnetic generator system (right); note that the size scale is similar, and that both involve a transfer mechanism . .3 2.1 Schematic for generic thermal-electric energy harvesting device. .6 2.2 Thermoelectric device relative efficiency as a function of figure of merit for various operating temperatures. .8 2.3 Plot of ideal efficiency for a Seebeck effect based thermoelectric device, as a function of operating temperature difference, with cold- side temperature at Tcold = 300K and Z = 1. 10 2.4 Reduced saturation magnetization of iron, nickel and cobalt, as a function of reduced temperature, for various values of net atomic angular momentum (Image borrowed from Cullity et.al. 2011 [CG11]). 15 2.5 Graphical technique for computing reduced magnetization for the case of non-zero applied field (Image borrowed from Cullity et.al. 2011 [CG11]); curve 1 represents the case of J = 1=2. 16 2.6 Bulk behavior of a ferromagnetic material in the vicinity of its Curie point (Image borrowed from Cullity et.al. 2011 [CG11]). 18 x 3.1 ANSY S R modeling of the magnetic force of attraction between gadolinium (top rectangle) and NdFeB (bottom rectangle). 26 3.2 Simulation results revealing an anomaly between magnetic force and gadolinium thickness. 27 3.3 Polishing process to determine gadolinium
Details
-
File Typepdf
-
Upload Time-
-
Content LanguagesEnglish
-
Upload UserAnonymous/Not logged-in
-
File Pages141 Page
-
File Size-