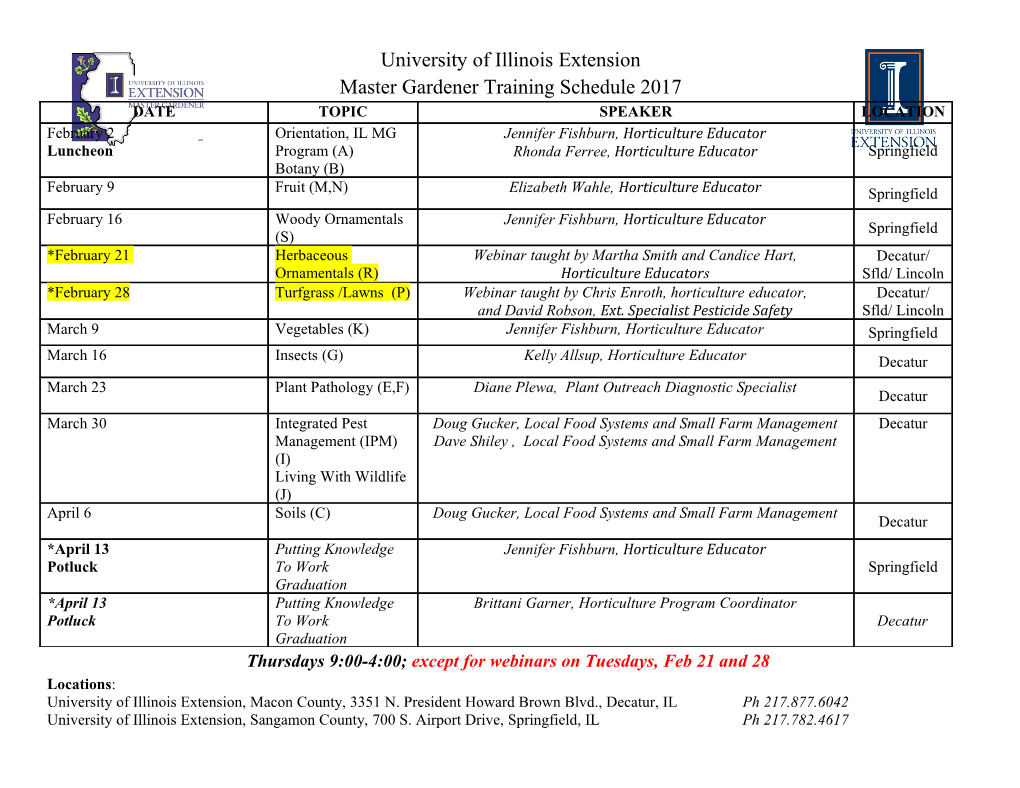
1 DRAFT 3/4/2011 2 Rainfall Simulation Studies – A Review of Designs, Performance and 3 Erosion Measurement Variability 4 5 Mark E. Grismer 6 Depts. of Hydrologic Sciences and Biological & Agricultural Engineering 7 UC Davis 8 9 Table of Contents pages 10 INTRODUCTION 1-2 11 12 EROSION PROCESSES – Model Development 2-16 13 Universal Soil Loss Equation (USLE) 4-8 14 Water Erosion & Prediction Project (WEPP) 8-12 15 Characterizing Erosion - Continued Developments 12-16 16 17 NATURAL & SIMULATED RAINFALL ENERGIES AND INTENSITIES 16-25 18 19 RAINFALL SIMULATOR (RS) DESIGNS 26-57 20 Basic RS Designs – Overview 26-29 21 Table AA. Summary of RS performance characteristics 29-34 22 Basic RS Designs –Drop sizes, Distribution & Intensity Uniformity 23 Drop-former type RSs 35-37 24 Spray-nozzle type RSs 37-46 25 Basic RS Designs –Drop-size Distribution & Intensity Effects on KEs 46-57 26 27 FIELD RS METHODOLOGIES – Effects of measurement methods & plot conditions 28 57-72 29 APPLICABILITY OF FIELD RS-DERIVED EROSION RATES – Up-scalability? 30 72-76 31 32 Recent Rainfall Simulation Studies in the Tahoe Basin 76-83 33 34 SUMMARY & CONCLUSIONS 83-87 35 36 REFERENCES 88-101 37 38 0 39 INTRODUCTION 40 The unpredictability, infrequent and random nature of natural rainfall makes 41 difficult the study of its effects on soils while rainfall is occurring. The use of rainfall 42 simulators (RSs) and perhaps runoff simulators for rill erosion can overcome some of 43 these difficulties, enabling a precise, defined storm centrally located over runoff 44 measurement “frames”. RSs are often used to study the effects of various soil factors on 45 rates of infiltration and erosion in the field. Following development of sprinkler and 46 drop-former designs in the 1950s, a variety of RSs have been developed for use in the 47 laboratory and field. Generally, these are associated with smaller plot sizes on the order 48 of 1 m2 and are directed at assessment of soil cover, tillage or practice treatment effects, 49 determination of soil inter-rill and rill erodibilities for model parameterization, evaluation 50 of pollutant transport or dispersal rates and other applications of particular interest to the 51 research group. That no standardized methodology has been proposed or can be 52 identified in the literature, making comparisons between study results difficult has long 53 been recognized. Such efforts in Europe were represented in part by conferences and 54 meetings resulting in a special journal issue (Parsons and Lascelles, 2006) that detailed 55 some of the efforts of a working group having the goals of cataloging the RSs in use, 56 their specifications and performance characteristics, as well as developing a standard RS 57 evaluation and test methodology for broad use such that data collected by various studies 58 can be compared. Agassi and Bradford (1999) completed a review of inter-rill erosion 59 measurement studies using rainfall simulation methods and categorized the 60 “methodology problems” into inadequate characterization of (a) the type of RS its rainfall 61 intensities, mean drop size and drop-size distribution, and water quality deployed, (b) the 62 soil plot physical and chemical properties, and (c) the type of results obtained and how 63 they are presented. Kinnell (2005, 2006) completed thorough reviews of the processes 64 associated with raindrop impacted erosion and noted that both conceptual models and 65 measurements fail in various respects to adequately characterize observed erosion 66 processes from bare soils. Concerns such as these have also arisen in the Tahoe Basin, 67 because a variety of methods for measurement of infiltration and erosion rates have been 68 deployed, but comparisons between results of different studies are uncertain. 1 69 The objective of this paper is to review the more recent literature of the past two 70 decades concerning application of RS techniques in the field and how they might apply to 71 forested, rangeland, and ski-run conditions similar to that found in the Sierra Nevada. As 72 many of the RS-derived erosion measurement efforts are, at least in part, motivated by 73 the historical conceptual view of erosion processes, first, the prevailing descriptions of 74 the erosion processes as they developed from the classic USLE-based interpretation to 75 sediment transport and WEPP-based analyses are considered. Next, as a primary concern 76 of the past has been the ability of RSs to replicate “natural” rainfall characteristics, 77 available studies of these characteristics are reviewed and compared with laboratory 78 analyses of rain drop-sizes, their distributions and kinetic energies (KEs). These reviews 79 set the stage for consideration of RS designs and field methodologies as they may have 80 been affected by attempted definitions of erodibility and “natural” rain characteristics. 81 Following review of RS designs and issues associated with field plot conditions, some of 82 the key issues associated with RS-based erosion measurements; the processes involved in 83 forested landscapes, their interpretation, sources of error or uncertainty and up-scaling 84 plot results to hillslope and catchments are considered. Here, the focus is largely on 85 “portable” RS usable in field studies of these various processes on a range of slopes. 86 87 88 EROSION PROCESSES – USLE and WEPP Development 89 The rainfall runoff and erosion process is usually considered to be initiated with 90 rain drop impact on bare or nearly bare soils, detaching and splashing soil particles and 91 subsequent downslope transport as part of overland flow (Mutchler et al, 1988). Raindrop 92 momentum or kinetic energy (KE) is a product of raindrop size (mass) and velocity or 93 velocity -squared at impact. Though Wischmeier and others originally found from 94 statistical analyses that rainfall KE alone was insufficient to describe erosivity, Lal 95 (1988) opined that it is a major factor in the soil detachment process, and likewise that 96 the total energy load of a storm is proportional to its erosivity. Net erosion rates 97 (sediment mass/unit area) are a function of both rainsplash and overland flow transport. 98 For shallow slopes, rainsplash is considered the dominant factor in causing erosion; as the 99 slope angle increases, runoff becomes the dominant factor (Kamalu, 1994). Splash 2 100 erosion alone does not redistribute large amounts of soil, rather it serves to detach soil 101 material for transport by runoff (Evans, 1980). Runoff, as interrill overland flow, carries 102 with it the smaller detached particles, and acts to remove the most erodible silt and very 103 fine sand particles from the soil surface as it flows downhill (Press and Siever, 1986). 104 When the rainfall intensity exceeds the infiltration rate, surface water excess 105 accumulates on the soil; when the surface depressions are filled, runoff in the form of 106 sheet overland flow can occur. Surface roughness reduces the velocity of overland flow 107 (Evans, 1980); differences in surface roughness can be due to soil textural variations, 108 tillage, residues on the surface, or the presence of living plant stems. Overland flow is a 109 very elusive and difficult process to measure, and as such, relatively little is known about 110 the actual mechanics of soil loss by this process (Emmett, 1980), though classic sediment 111 transport concepts are generally used. 112 Surface cover, in the form of living vegetation or residues, both reduces the 113 impact energy of the raindrops and prevents them from striking the soil surface. Raindrop 114 impacts both compact the surface and possibly detach surface soil particles from bare 115 soils; together these processes can seal the soil surface, reducing the infiltration rate. At 116 low cover levels, runoff and erosion rates are related to the area of bare ground, 117 increasing as the bare ground area increases. Vegetated soils also have greater structure 118 and aggregation, leading to higher infiltration rates (Evans, 1980). The effect of plant 119 canopy cover on reducing runoff and erosion in natural rangeland environments has been 120 demonstrated to be due primarily to the increased litter cover, soil macro-porosity, and 121 soil structure that occur due to the presence of canopy cover, rather than to the direct 122 interception of rainfall (Simanton et al., 1991). Similarly, rock cover tends to reduce 123 erosion rates proportional to the cover fraction. 124 Generally with all else equal, erosion rates increase as the slope angle increases 125 (Evans, 1980). As slope increases, overland flow velocities increase (Kloosterboer and 126 Eppink, 1989) such that the greater surface flow velocity increases both the erosive 127 power and the flow competence (i.e. “transport capacity”) to carry suspended sediments 128 (Press and Siever, 1986). The slope angle is also important in the splash erosion process; 129 as the angle steepness increases, more soil is splashed downhill (Evans, 1980). However, 3 130 the runoff component is the most sensitive to slope change; beyond some threshold 131 inclination, it becomes the dominant erosive process (Kamalu, 1994). 132 Though only briefly outlined above, it is clear that erosion from soil surfaces 133 involves several inter-related processes that in the field combine in complex spatial and 134 temporal variations such that results from different erosion studies are difficult to 135 compare. These processes can include particle (aggregate) breakdown, particle 136 detachment, related splash effects then particle suspension and transport as part of 137 overland flow or wind, particle filtration by covers or mulch layers, particle movement 138 into the soil profile and so on. Clearly, all these processes are controlled by basic 139 hydrologic phenomena such as precipitation form and rates, soil infiltration rates and 140 capacity and the surface conditions (e.g.
Details
-
File Typepdf
-
Upload Time-
-
Content LanguagesEnglish
-
Upload UserAnonymous/Not logged-in
-
File Pages102 Page
-
File Size-