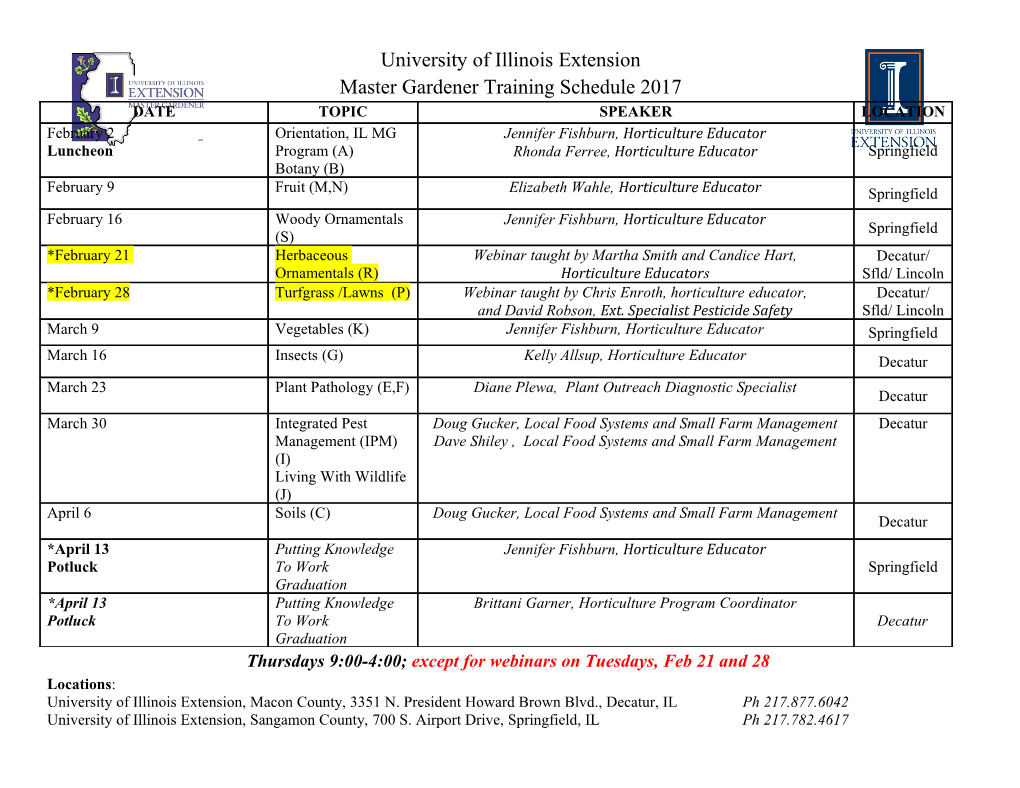
The Physics of Supernova 1987A Richard McCray Abstract We describe multiwavelength observations of the evolving spectra and images of Supernova (SN) 1987A, and we review the principles used to infer the physical conditions in the explosion debris. We interpret the early optical and gamma- ray light curves with a simple diffusion model. We review the evidence for dust formation in the debris. We show X-ray and optical observations that enable us to characterize and map the shock fronts caused by of the interaction of the debris with circumstellar matter. We describe how observations of millimeter emission lines due to rotational transitions of CO and SiO enable us to map the distribution, masses, and temperatures of these molecules in the debris. Contents 1 Introduction.............................................................. 2 2 Supernova Energetics...................................................... 3 3 The Light Curve........................................................... 4 4 X-Rays and Gamma Rays................................................... 6 5 Spectral Evolution......................................................... 7 6 Dust Formation........................................................... 8 7 Circumstellar Matter....................................................... 9 8 The Impact............................................................... 10 8.1 Plane-Parallel Shocks................................................. 10 8.2 Blast Wave and Reverse Shocks........................................ 12 9 Radiation from Shocked Gas................................................ 14 9.1 X-Ray Emission from Non-radiative Shocks.............................. 14 9.2 Radiative Shocks: The Hotspots........................................ 19 9.3 Balmer-Dominated Shocks: The Reverse Shock........................... 20 9.4 Doppler Tomography................................................. 21 10 Interior Debris............................................................ 23 10.1 Molecular Emission from Inner Debris................................... 23 10.2 Rotational Transitions of CO and SiO.................................... 25 R. McCray () Department of Astronomy, University of California, Berkeley, CA, USA e-mail: [email protected] © Springer International Publishing AG 2017 1 A.W. Alsabti, P. Murdin (eds.), Handbook of Supernovae, DOI 10.1007/978-3-319-20794-0_96-1 2 R. McCray 10.3 Modeling the Spectral Line Energy Distribution........................... 26 Cross-References.............................................................. 29 References................................................................... 29 1Introduction Supernova 1987A in the Large Magellanic Cloud was first observed on February 23, 1987. It is the brightest supernova since Kepler’s supernova of 1604. By virtue of its proximity, SN is the first supernova to be observed at every band of the electromagnetic spectrum and the first to be observed through its initial flash of neutrinos. SN1987A was classified as a Type II supernova, i.e., its spectrum was dominated by hydrogen lines. But it had an unusual light curve, which continued to brighten for about three months after its initial outburst before it began to fade. We now understand that this behavior was a consequence of the fact that its progenitor was a blue giant rather than a red giant star. Modern supernova surveys show that roughly 1–2% of Type II supernovae display light curves and spectral evolution similar to SN1987A. SN1987A is surrounded by a system of three circumstellar rings (Fig. 1), which evidently were ejected by the progenitor some 20,000 years before the supernova outburst. The cylindrical symmetry of the ring system strongly indicates that the progenitor of SN1987A was a binary star system. The absence of any evidence for a surviving companion star suggests that the two stars merged before the explosion, Fig. 1 SN1987A as seen with the Hubble Space Telescope in 2010 (Courtesy of Peter Challis) The Physics of Supernova 1987A 3 and this scenario may account for the facts that the progenitor was a blue giant and that it ejected the triple ring system. Today, almost 30 years after its discovery, SN1987A has made the transition to the supernova remnant phase, in which its luminosity is dominated by emission from shocks formed where the supernova blast wave encounters the inner circumstellar ring. Although the supernova debris has faded by a factor 107, it is still observable at wavelengths ranging from radio to gamma rays, and it continues to be the most intensively observed supernova in history. In this chapter, I aim to provide a simple framework for understanding the physics of this evolution. To do this, I will rely on rough order-of-magnitude estimates. The reader can find many references to the observations and more details of their interpretation in review articles by Arnett et al. (1989), McCray (1993) and McCray and Fransson (2016). 2 Supernova Energetics SN1987A is a core-collapse supernova, which means that the explosion is the result of the gravitational collapse of the iron core of a massive star. In the final stages of evolution, thermonuclear reactions in the core convert H to He, He to C, O, Ne, Si, and ultimately to Fe. Lacking any source of thermonuclear energy, the iron core cools and shrinks until it is supported by degeneracy pressure of electrons. At this point the core resembles a white dwarf star, having mass comparable to Mˇ, the mass of the Sun, and radius RC 1000 km. The mass of the core continues to increase as a result of thermonuclear reactions in shells surrounding the core. When the core mass exceeds MC D 1:4 Mˇ (the Chandrasekhar limit), electron degeneracy pressure can no longer withstand the pull of gravity. The core collapses 3=2 1=2 on the free-fall timescale, tff RC .GMC / 2 ms. The collapse is halted at a 15 3 radius Rcore 12 km, at which point the density of the core, core 10 gcm , is comparable to that of an atomic nucleus and the nuclear force becomes strongly 2 53 repulsive. The kinetic energy of infall, EG .3=5/GMC =Rcore 3 10 ergs, is converted to heat, which is divided equally among photons, electrons, positrons, and three species of neutrinos and antineutrinos. The temperature of the resulting fireball 4 3 can be estimated from the relationship EG D .43=8/aT Œ4Rcore=3, which yields 3=4 kT 100 MeVŒRcore=10 km . At such a temperature and density, the iron nuclei dissolve into neutrons and protons. Most of the protons are converted to neutrons by inverse beta decay. The result is a nascent neutron star. During the first few seconds after the collapse is halted, the hot neutron star is opaque, even to neutrinos. The neutrinos carry the internal heat by convection to the neutrinosphere, the surface above which the neutrinos can freely stream outward. The convection is violently unstable, and this instability reverses the infall of matter into the neutron star and deposits ESN 1%ofEG as thermal and kinetic energy of outflowing matter. This outflowing matter acts as a piston, driving a shock wave through the envelope of the star. Thermonuclear reactions in the shocked gas synthesize heavy elements, including the radioisotopes 56Ni, 57Ni, and 44Ti. 4 R. McCray Hydrodynamic instabilities during the first few days after the explosion cause the heavy elements to be mixed with the O, C, Ne, Si, etc., that were synthesized during the late stages of evolution of the progenitor star and with the hydrogen/helium of the stellar envelope. This mixing is macroscopic, not microscopic, in the sense that the fragments of different chemical composition do not interact chemically except at their boundaries. Most of the binding energy of the newly formed neutron star emerges as a burst of neutrinos lasting several seconds. In the case of SN1987A, this neutrino flash was detected through flashes of Cerenkov light seen in deep underground tanks of water in Japan and Ohio. The total energy of the neutrino flash was E 5 52 10 ergs, as expected for the formation of a neutron star. (E D EG =6, because the gravitational collapse energy was divided equally among six types of neutrinos, only one of which was detected). The duration, 10 s, and the characteristic temperature of the neutrinos, kT 4 MeV, were also just as predicted for the formation of a neutron star. As mentioned, a small fraction of the energy of the neutrinos is deposited as thermal and kinetic energy in a hot bubble of gas that reverses the flow of matter falling toward the nascent neutron star. This bubble, which resides within the neutrinosphere at 100 km, drives a shock wave through the envelope of the star. The passage of the shock deposits half the energy of the bubble as kinetic energy of the exploding star and half as thermal energy. The thermal energy resides mostly as photons. One can estimate the temperature of the radiation by equating 3 4 51 7 3 1=4 .4R=3/aT0 D 1:5 10 ergs, which gives T0 10 K ŒE51=R12 , where 51 12 E51 D ESN =10 ergs and R12 is the radius of the progenitor in units 10 cm. We may estimate the characteristic expansion velocity, V , of the supernova 2 debris by equating the explosion energy, ESN D MV =2, which yields V D 1=2 1 3200ŒE51=M10 km s , where M10 is the debris mass in units of 10 solar masses. The time for the supernova blast to propagate through the debris is given by 1=2 1=2 t0 D R=V 1 h R12M10 E51 . 3 The Light Curve Figure 2 shows light curves of various components of SN1987A. The optical display of the supernova will commence when the blast arrives at the photosphere, an event called shock breakout. According to the (very rough) estimate above, this event should occur about 1 h after the neutrino flash. At shock 6 breakout, the temperature of the photosphere will suddenly rise to TS 10 K and subsequently decrease rapidly (within a few hours) as the debris expands. The shock breakout yields a flash of ionizing radiation having initial luminosity 45 L0 10 ergs=s and total fluence of ionizing (>13:6 eV) radiation Fi D 2 1057 photons. The net energy of ionizing radiation released at shock breakout, 47 Ei 10 ergs, is negligible compared to the total energy of the supernova explosion, 51 ESN 3 10 ergs.
Details
-
File Typepdf
-
Upload Time-
-
Content LanguagesEnglish
-
Upload UserAnonymous/Not logged-in
-
File Pages30 Page
-
File Size-