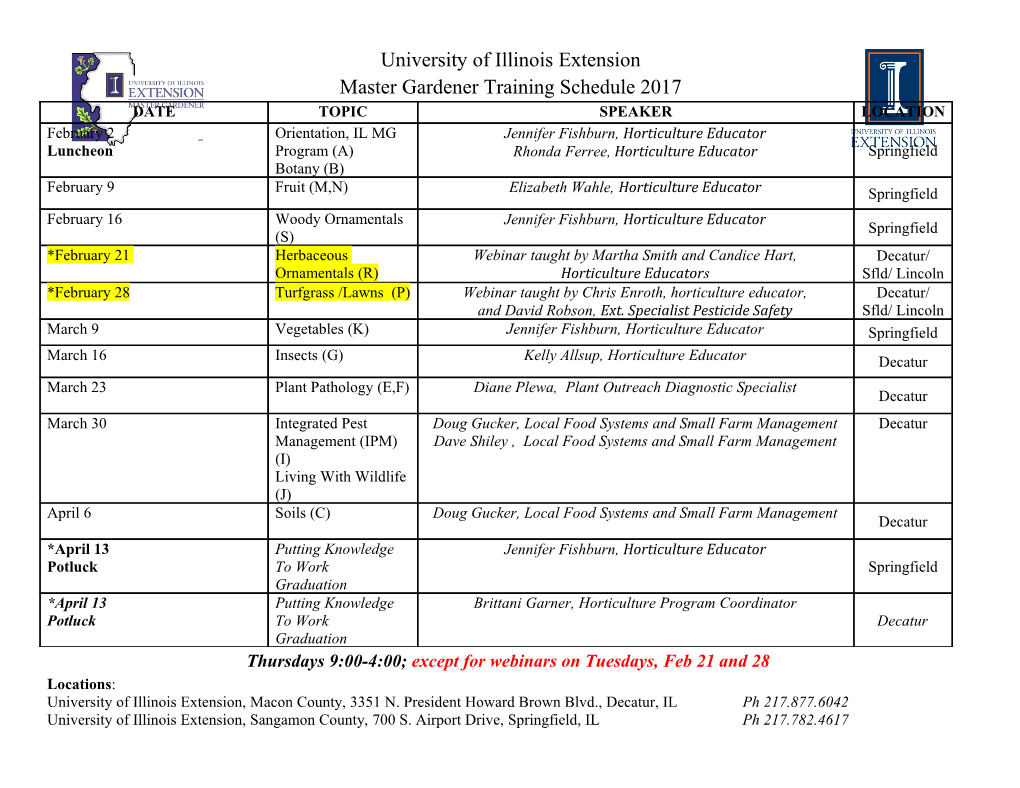
PhD Thesis OPTIMAL PERFORMANCE ANALYSIS OF A SOLAR THERMAL ENERGY STORAGE PLANT BASED ON LIQUID AMMONIA Submitted by Engr. Sadaf Siddiq (08F-UET/PhD-ME-47) Supervised by Prof. Dr. Shahab Khushnood Department of Mechanical Engineering Faculty of Mechanical and Aeronautical Engineering University of Engineering and Technology Taxila, Pakistan July 2013 OPTIMAL PERFORMANCE ANALYSIS OF A SOLAR THERMAL ENERGY STORAGE PLANT BASED ON LIQUID AMMONIA by Engr. Sadaf Siddiq (08F-UET/PhD-ME-47) A proposal submitted for research leading to the degree of Doctor of Philosophy in MECHANICAL ENGINEERING Approved by External Examiners ________________________________ ________________________________ (Engr. Dr. M. Javed Hyder) (Engr. Dr. Ejaz M. Shahid) Dean of Engineering, Associate Professor, Pakistan Institute of Engineering & Applied Sciences Department of Mechanical Engineering, Nilore, Islamabad. University of Engineering & Technology, Lahore. Internal Examiner (Research Supervisor) ________________________________ (Engr. Dr. Shahab Khushnood) Professor, Department of Mechanical Engineering, University of Engineering & Technology, Taxila. Department of Mechanical Engineering Faculty of Mechanical and Aeronautical Engineering University of Engineering & Technology Taxila, Pakistan. ii DECLARATION I declare that all material in this thesis is my own work and that which is not, has been identified and appropriately referenced. No material in this work has been submitted or approved for the award of a degree by this or any other university. Signature: _____________________________ Author’s Name: ________________________ It is certified that the work in this thesis is carried out and completed under my supervision. Supervisor: Prof. Dr. Shahab Khushnood Department of Mechanical Engineering Faculty of Mechanical and Aeronautical Engineering University of Engineering and Technology Taxila, Pakistan. iii ABSTRACT This work focuses on extending the use of a solar thermal energy plant from an intermittent energy source to a base load power plant by incorporating an efficient thermal storage feature. A reference 10 MWe solar thermal plant design is considered with liquid ammonia as a working fluid for energy production, in a Rankine Cycle, as well as a thermal storage medium. During periods of no solar insolence, the recovery system, based on an industrial ammonia synthesis system, is used to drive the power conversion unit and enable continuous operation. A thermofluid model, based on the continuity, momentum and energy conservation equations, is used to carry out a numerical simulation of the plant, to determine the process variables and subsequently carry out an integrated plant energy recovery analysis. The objective of this work is to maximize the efficiency of the plant by a detailed consideration of the most critical process in the plant: the energy recovery unit. This is carried out by (i) estimating the sensitivity of non-uniform catalyst concentration in a synthesis reactor, and (ii) obtaining an optimal configuration from a variational Lagrangian cost functional and applying Pontryagin’s Maximum Principle. The optimal configuration is used to recommend a re- design of the synthesis reactor and to quantify the energy recovery benefits emanating from such a recommendation. Industrial optimal configurations are achieved by carrying out the analysis with the simulation code, Aspen Plus™, to design a heat removal system surrounding the catalyst beds, and incorporating the effect of standard industrial processes iv such as purge gas removal, quench gas recycling, and recycle ratio to achieve the optimal temperature profile obtained for the synthesis reactor considered in this work. This work quantifies the maximum energy recovery in a base-load solar thermal plant utilizing the existing environment of chemical process industry. It is concluded that a one- dimensional model, with mass and energy conservation equations using the Temkin-Pyzhev activity and pressure-based kinetics rate expressions, predicted an optimal ammonia conversion of 0.2137 with a thermal energy availability of 20 MWth. A comprehensive process simulation using Aspen Plus™ predicts an optimal ammonia conversion of 0.2762 mole fraction at exit, with two inter-bed heat exchangers having optimal temperature drops of 205K and 95K respectively, and yielding a thermal availability of 45.6 MWth. The thermal energy availability of a base-load solar thermal plant can be increased by 15% in the ammonia conversion and over 25% in thermal energy availability for energy recovery. v To my family . vi ACKNOWLEDGEMENTS During the development of my PhD studies at University of Engineering & Technology Taxila, several persons and institutions collaborated directly and indirectly with my research. Without their support it would be impossible for me to finish my work. That is why I wish to dedicate this section to recognize their support. I want to start expressing a sincere acknowledgement to my advisor, Prof. Dr. Shahab Khushnood because he gave me the opportunity to research under his kind guidance and supervision. I received motivation; encouragement and support from him during all my studies. I owe Special thanks to Dr. Zafar Ullah Koreshi for the his support, guidance, and transmitted knowledge for the completion of my work. With him, I have learned writing papers for conferences and journals and sharing my ideas with the scientific community. I also want to thank the example, motivation, inspiration and support I received from Dr. Tasneem M. Shah, Dr. Arshad H. Qureshi and Dr. M. Bilal Khan. The Grant from University of Engineering & Technology Taxila provided the funding and resources for the development of this research and validation of my work. At last, but the most important I would like to thank my family, for their unconditional support, inspiration, love and prayers. vii NOMENCLATURE A Cross-sectional area (m 2) ANU Austrailian National University -1 -1 C p Specific heat at constant pressure (kJ kmol K ) C r Compression Ratio CSP Concentrating Solar Power E Activation energy (kJ kmol -1) F →sf Force (external, fluid to solid) 0 -1 FN Initial nitrogen molar flow rate (kmol h ) Gt Giga-ton (10 9 ton) Η Hamiltonian Ηˆ Enthalpy per unit mass J Functional * J i , J i Molar Fluxes K Kinetic Energy Ka Equilibrium constant KBR Kellogg Brown and Root™ L Length of synthesis reactor (m) MTD Metric tonnes per day Mtoe Million ton of oil equivalent MWe Megawatt electric MWth Megawatt thermal OEM One Equation Model P Pressure (MPa) Ρ Linear Momentum PMP Pontryagin’s Maximum Principle PV PhotoVoltaic Q Heat viii R Universal gas constant 8.3144 kJ kmol -1 K-1 -1 -3 R A Reaction rate (kmol NH 3 h m catalyst) RK-4 4th order Runge-Kutta S Surface Area (m 2) T Temperature (K) TEM Two Equation Model TSP Thermal Storage Plant TWh Terawatt-hours (10 12 Watt-hrs) U Internal Energy (kJ) Uˆ Internal Energy per unit mass W Watts ai Activity for specie i c Total Molar Concentration ci Molar Concentration of Specie i dp Particle Diameter g Gravitational acceleration (9.81 ms -2) * ji , ji Mass Fluxes kWe kilowatt Electric kW chem. kilowatt chemical kW th kilowatt thermal m Mass (kg) 0 -1 ni Initial mole flow rate of specie i (kmol h ) ppm Parts per million r Molar Production t Time u Control variable v Velocity (m s -1) w Work x Distance along catalyst bed (m) ix x′ Normalized Distance along catalyst bed (m) yi Mole fraction for specie i o yi Initial Mole fraction for specie i , zz N Fractional conversion of Nitrogen Greek ∆ -1 H r Heat of reaction (kJ kmol NH 3) ε Extent of reaction Φ Potential Energy φ i Fugacity coefficient for specie i ω Mass Flow Rate (kghr -1) τ Shear Stress η Catalyst effectiveness factor ψ The void space of the bed λ Lagrange multiplier ξ x)( Catalyst spatial factor θ (x′) Optimal Temperature ρ Density (kg m -3) r σ Vector containing state variables Subscripts eqm Equilibrium i Species in a multi component system, i = 4,3,2,1 ,.... N opt Optimal s Isentropic tot Total amount of entity in a macroscopic system 0 Evaluated at a surface 2,1 Evaluated at cross sections 1 and 2 x Table of Contents ABSTRACT ....................................................................................................................................................... IV ACKNOWLEDGEMENTS ............................................................................................................................ VII NOMENCLATURE ...................................................................................................................................... VIII TABLE OF CONTENTS .................................................................................................................................. XI TABLE LIST ................................................................................................................................................... XIII FIGURE LIST ................................................................................................................................................ XIV 1 INTRODUCTION ..................................................................................................................................... 1 1.1 SOLAR ENERGY : POTENTIAL AS A RENEWABLE ENERGY SOURCE ............................................................ 2 1.2 SOLAR POWER PLANTS IN OPERATION ...................................................................................................... 5 1.2.1 PV Plants .......................................................................................................................................
Details
-
File Typepdf
-
Upload Time-
-
Content LanguagesEnglish
-
Upload UserAnonymous/Not logged-in
-
File Pages206 Page
-
File Size-