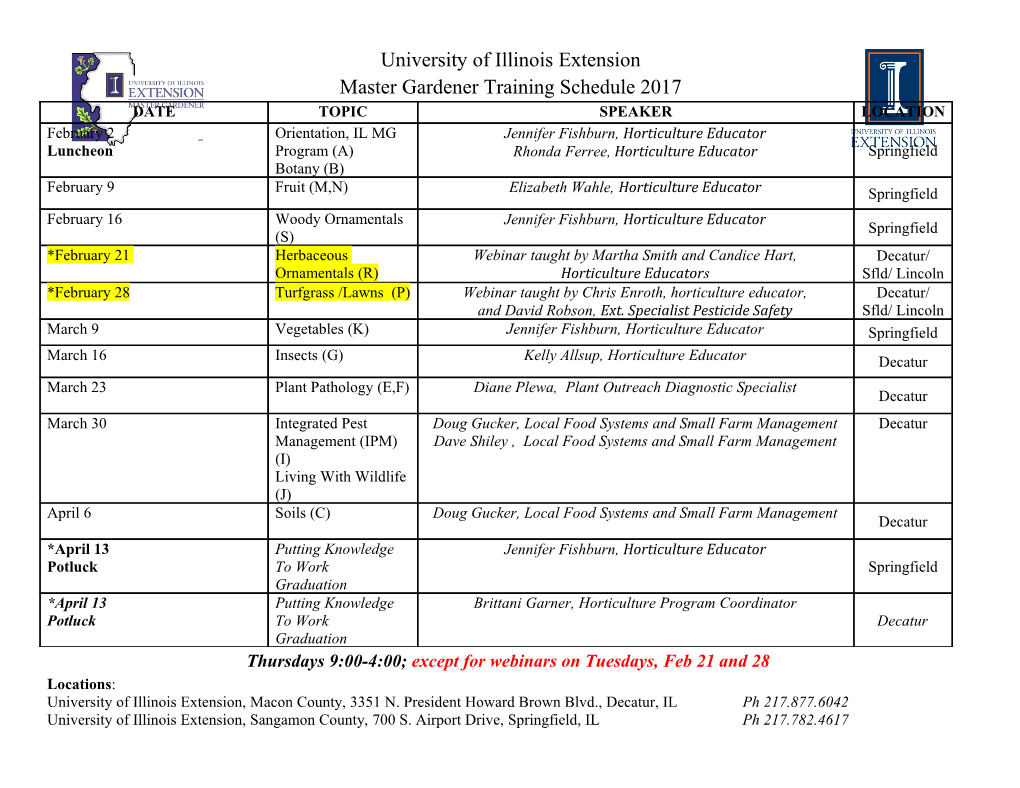
The evolution of close binaries with white dwarf components Proefschrift ter verkrijging van de graad van doctor aan de Radboud Universiteit Nijmegen op gezag van de rector magnificus prof. mr. S.C.J.J. Kortmann, volgens besluit van het college van decanen in het openbaar te verdedigen op maandag 7 oktober 2013 om 12.30 uur precies door Silvia Gerarda Martina Toonen geboren op 31 mei 1984 te Nijmegen Promotoren: Prof. dr. G. Nelemans KU Leuven Prof. dr. P.J. Groot Manuscriptcommissie: Prof. dr. N. de Groot Prof. dr. B.T. Gaensicke University of Warwick Prof. dr. D. Maoz Tel-Aviv University Prof. dr. S. Portegies Zwart Leiden University dr. O.R. Pols c 2013, Silvia Toonen The evolution of close binaries with white dwarf components Thesis, Radboud University Nijmegen Illustrated; with bibliographic information and Dutch summary ISBN: 978-94-6191-884-0 Optimism is the faith that leads to achievement. Nothing can be done without hope and confidence. Helen Keller Contents 1 Introduction 1 1.1 Stars....................................... 1 1.2 Stellar evolution of low- and intermediate-mass stars . ........... 1 1.3 Binaryevolution ................................ 2 1.3.1 Rochelobegeometry .......................... 2 1.3.2 Masstransfer .............................. 4 1.3.3 Accretionontowhitedwarfs . 5 1.3.4 Common-envelopeevolution . 6 1.4 SupernovaeTypeIa............................... 7 1.5 Binarypopulationsynthesis . ... 8 1.6 Thisthesis.................................... 9 2 The effect of common-envelope evolution on the visible population of post-common-envelope binaries 13 2.1 Introduction................................... 14 2.2 Method ..................................... 15 2.2.1 SeBa - a fast stellar and binary evolution code . ..... 15 2.2.2 Theinitialstellarpopulation. ... 16 2.2.3 Common-envelopeevolution . 16 2.2.4 Galacticmodel ............................. 17 2.2.5 Magnitudes ............................... 17 2.2.6 Selectioneffects............................. 18 2.3 Results...................................... 18 2.3.1 TheSDSSPCEBsample........................ 25 2.3.2 VariableCEefficiency ......................... 31 2.4 Discussionandconclusion . .. 32 2.A Population synthesis code SeBa . ... 35 i Contents 2.B Thespectraltype-massrelation . ... 36 3 The single degenerate supernova type Ia progenitors: Studying the influ- ence of different mass retention efficiencies 39 3.1 Introduction................................... 40 3.2 Evolution of single degenerate SNIa progenitors . ......... 42 3.2.1 Globalevolution ............................ 42 3.2.2 Commonenvelopephase . 43 3.2.3 Growthofthewhitedwarf . 45 3.3 Method ..................................... 47 3.3.1 Retentionefficiencies .. .. .. 48 3.3.2 Islands.................................. 49 3.4 Results: SNIa rates and delay times . .... 51 3.5 Discussion.................................... 54 3.6 Conclusions ................................... 55 3.A Retentionefficiencies . .. .. .. 57 3.A.1 Retention efficiencies based on Nomoto et al. 2007 . ..... 57 3.A.2 Retention efficiencies based on Ruiter et al. 2009 . ..... 58 3.A.3 Retention efficiencies based on Yungelson 2010 . ..... 59 4 The influence of mass-transfer variability on the growth of the mass of white dwarfs in accreting systems 61 4.1 introduction................................... 62 4.2 Mass-transfervariability . .... 63 4.2.1 Theoreticalconsiderations . .. 64 4.2.2 Observations .............................. 65 4.3 Model ...................................... 66 4.3.1 Mass-transfervariability . .. 66 4.3.2 Integrated retention efficiency . .. 69 4.4 Application to binary stellar evolution . ....... 71 4.5 BinarypopulationSynthesis . ... 74 4.6 Resultsanddiscussion . .. .. .. 75 4.7 Conclusions ................................... 77 5 Supernova Type Ia progenitors from merging double white dwarfs: Using a new population synthesis model 83 5.1 Introduction................................... 84 5.2 SeBa - a fast stellar and binary evolution code . ....... 87 5.2.1 Impact on the population of double white dwarfs . .... 88 5.3 Two models for common-envelope evolution . ..... 90 ii Contents 5.3.1 Impact on the population of double white dwarfs and type Ia super- novaprogenitors ............................ 91 5.4 Evolutionary paths to supernova type Ia from the double degenerate channel 93 5.4.1 common-envelopechannel . 95 5.4.2 Stablemasstransferchannel . 97 5.4.3 Formationreversalchannel. 99 5.5 Delaytimedistribution. .. 99 5.6 Population of merging double white dwarfs . ...... 103 5.7 Conclusion and discussion . 107 5.A Most important changes to the population synthesis code SeBa. 110 5.A.1 Treatmentofwindmass-loss . 110 5.A.2 Accretionontostellarobjects . 112 5.A.3 Stabilityofmasstransfer. 115 6 PopCORN: Hunting down the differences between binary population syn- thesis codes 121 6.1 Introduction................................... 122 6.2 Binaryevolution ................................ 124 6.2.1 Rochelobeoverflow. .. .. .. 124 6.3 Binary population synthesis codes . ..... 128 6.3.1 binary_c/nucsyn ............................ 128 6.3.2 TheBrusselscode............................ 129 6.3.3 SeBa................................... 130 6.3.4 StarTrack ................................ 130 6.4 Method ..................................... 131 6.4.1 Assumptionsforthisproject . 132 6.4.2 Normalisation.............................. 134 6.5 Results...................................... 135 6.5.1 Singlewhitedwarfsystems. 135 6.5.2 Doublewhitedwarfs .. .. .. 170 6.6 Summary of critical assumptions in BPS studies . ....... 184 6.7 Conclusion.................................... 187 6.A BackbonesoftheBPScodes . 189 6.A.1 Singlestarprescriptions . 191 6.A.2 Stabilityofmasstransfer. 191 6.A.3 Stablemasstransfer .. .. .. 193 6.A.4 Windmassloss ............................. 194 6.A.5 Angular momentum loss from winds . 195 6.A.6 Evolutionofheliumstars. 195 6.A.7 Generating the initial stellar population . ...... 196 iii Contents 6.B Typical variable assumptions in BPS codes . ...... 196 6.B.1 Accretionefficiency . .. .. .. 198 6.B.2 Angular momentum loss during RLOF . 198 6.B.3 Commonenvelopeevolution . 199 6.B.4 Windaccretion ............................. 200 6.B.5 Tides................................... 200 6.B.6 Magneticbraking ............................ 200 6.B.7 Initialpopulation ............................ 201 Bibliography 203 Summary 213 Chapter 2: Common-envelope evolution . ..... 213 Chapter 3: Mass retention efficiency of accreting white dwarfs .......... 214 Chapter 4: Mass transfer variability towards accreting whitedwarfs . 215 Chapter 5: Double white dwarf binaries as supernovae Type Ia progenitors . 215 Chapter 6: Binary population synthesis . ...... 216 Conclusion....................................... 217 Samenvatting 219 Introductie ...................................... 219 Hetlevenvaneenster... ............................ 219 ..metzijntweeën................................ 220 SupernovaevantypeIa............................. 223 Ditproefschrift .................................... 223 Hoofdstuk 2: Instabiele massa-overdracht . ..... 225 Hoofdstuk 3: Hoe efficiënt kan een witte dwerg groeien door accretie?................................. 226 Hoofdstuk 4: Variabiliteit van de snelheid van massa-overdracht naar witte dwergen ................................. 226 Hoofdstuk 5: Supernovae van type Ia veroorzaakt door samensmeltende dubbelewittedwergen . 227 Hoofdstuk 6: Het simuleren van dubbelsterpopulaties . ....... 228 Curriculum vitæ 229 List of Publications 231 Acknowledgements 233 iv Chapter 1 Introduction 1.1 Stars A star is a sphere of gas and plasma that holds its shape through hydrostatic and thermal equilibrium. Hydrostatic equilibrium in a star is achieved by a balance between the force of gravity and the pressure gradient force. The force of gravity pushes the stellar material towards the center of the star, while the gas pressure pushes the material outwards into space. The combination of the pressure, density, temperature and chemical composition enforces that stars are gaseous throughout. When a star is in thermal equilibrium, the tem- perature of the gas is constant over time for a given radius. The temperature is maintained by energy production inside the star. Many energy sources are available, e.g. gravitational, chemical and thermonuclear energy, but only thermonuclear energy can account for the stellar luminosities and lifetimes. In a thermonuclear reaction (‘burning’), energy is pro- duced by the fusing of two nuclei into a more massive nucleus. Energy losses at the surfaces of stars is what we as observers on Earth perceive as starlight. 1.2 Stellar evolution of low- and intermediate-mass stars The equilibrium structure of a star slowly (and sometimes rapidly) changes in time as the energy production in the star changes. The long-term evolution of the star is driven by successive phases of nuclear burning. Initially stars mainly consist of hydrogen, which is fused into helium in the stellar core. This phase is known as the main-sequence phase. It 10 −2.8 lasts for about 90% of the stellar lifetime, which is approximately 10 (M/M⊙) yr, where 1 M is the mass of the star and M⊙ the mass of the Sun . When hydrogen is exhausted in the core, the star increases in size and becomes a giant. Core burning ceases, however, 1This is called the nuclear evolution timescale. 1 Chapter 1 : Introduction hydrogen burning continues in a shell around the inert core. Since the core is no longer supported by enough
Details
-
File Typepdf
-
Upload Time-
-
Content LanguagesEnglish
-
Upload UserAnonymous/Not logged-in
-
File Pages242 Page
-
File Size-