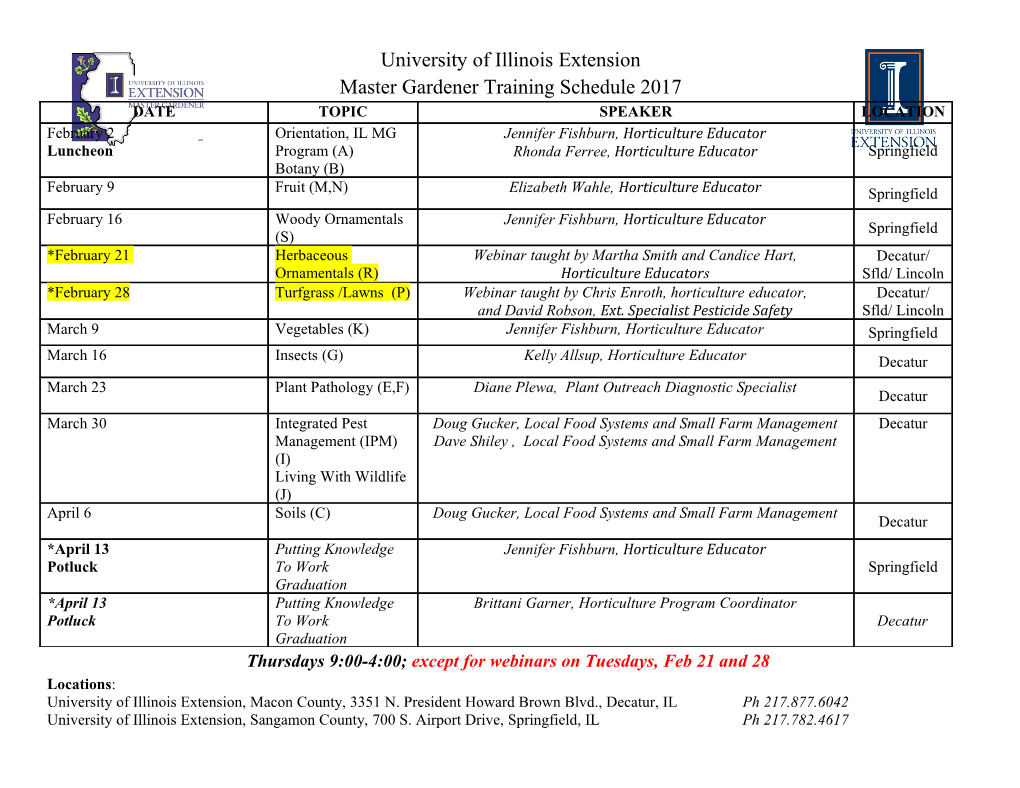
2 Nucleation Marco Mazzotti,1 Thomas Vetter,2 David R. Ochsenbein,1 Giovanni M. Maggioni,1 Christian Lindenberg3 2.1 Introduction The term “nucleation” is used to describe the onset of the formation of a new phase from a parent phase [1, 2]. Examples of nucleation processes include the formation of vapor bubbles in a liquid phase, the formation of droplets from a vapor phase or from another liquid, as well as the formation of crystalline particles from vapor, liquid or even another solid. In the following, we will focus on the formation of new crystalline particles from solution exclusively. Apart from the breakage of an already existing particle into two or more pieces, nucleation is the only mechanism generating new crystals and is therefore of fundamental interest. From a processing perspective it is notoriously a phenomenon hard to control in batch crystallizers, a fact that increases the importance of process design strategies that avoid or minimize nucleation through the addition of previously prepared seed crystals. It is useful to classify the events leading to the formation of nuclei highly anisotropic into different types [3, 4]: primary homogeneous nucleation refers to the formation of nuclei from clear liquids; primary heterogeneous nucleation occurs when nuclei are formed with the participation of foreign surfaces (such as stirrers, crystallizer walls, or crystals of another form); secondary nucleation describes the formation of nuclei of one crystal form with the participation of already-present crystals of the same crystal form. While much of what is 1Institute of Process Engineering, ETH Zürich, Switzerland 2School of Chemical Engineering and Analytical Science, University of Manchester, United Kingdom 3Novartis Pharma AG, Switzerland 1 CHAPTER 2. NUCLEATION described in the following sections applies to all three types of nucleation, there are slight differences that need to be accounted for. It is noteworthy that these different nucleation mechanisms can occur simulta- neously and to varying degrees throughout a crystallization process; moreover, the nucleation rates of different solids, e.g., polymorphs, may compete with each other. For instance, starting from a clear solution containing a solute, it is possible that mixtures of different polymorphs are obtained during the crystallization process when the nucleation kinetics of the different crystal forms are comparable. However, since different crystal forms exhibit different stability, all forms but the most stable one, will ultimately convert into the stable form upon reaching thermodynamic equilibrium. Nevertheless, all pathways for nucleation share the fact that the formation of nuclei involves the concomitant creation of a new bulk phase and of an interface. When there is a driving force for crystallization, the former leads to a decrease in free energy of the system, while the latter increases it. This interplay of contributions often leads to the presence of a substantial energy barrier for the creation of a nucleus. In such cases, the original state of the parent phase is not thermodynamically unstable, but rather metastable and the rate of formation of nuclei, i.e., the nucleation rate, is finite. This implies that a purely thermodynamic understanding of the system, albeit essential, is insufficient to describe nucleation; a kinetic understanding of the system is additionally required in order to answer questions pertaining to the dynamics of the system. Furthermore, the effect of the operating conditions on both aspects needs to be understood. Theories of nucleation aim at achieving this goal and a brief overview of the most important theoretical frameworks, experimental characterization tools, and applications shall be given in the following. Finally, this chapter will also highlight how the use of seed crystals in a crystallization process allows obtaining a desired crystal form, which is of importance when the performance of a product strongly depends on the manufactured crystal form. 2.2 Homogeneous Nucleation In order to describe the formation of nuclei, we must first define the driving force for crystallization. We can express it as the difference in chemical potential between a solute molecule in the solution at its current state, µ`, and in the ∗ solution’s equilibrium state, µ` . In practical applications, it is more convenient 2 2.2. Homogeneous Nucleation to express this driving force in terms of the supersaturation S. These quantities can be related by: ∗ a` c ∆µ = µ` − µ` = kT ln S = kT ln ∗ ≈ kT ln ∗ (2.1) a` c where k is the Boltzmann constant, T is the temperature, a` represents the ∗ activity of the solute in the supersaturated solution and a` is the activity of the solute in the solution’s equilibrium state (note that al = cγ, where c is the concentration and γ its activity coefficient). While the last step introduced in Eq. (2.1) using concentrations instead of activities is only accurate for ideal solutions, where γ ≈ γ∗ ≈ 1, or when γ/γ∗ ≈ 1 (which is a much less stringent requirement), it represents a useful approximation as concentrations are experimentally accessible quantities. Concerning the thermodynamics and kinetics of homogeneous nucleation, two theories are of particular importance. The first one is the oldest and probably best known theory of nucleation: classical nucleation theory (CNT). Originally developed for the nucleation of droplets and bubbles, the CNT is also extensively applied to crystals, which, in stark contrast to droplets/bubbles, exhibit a strong supramolecular structure. The second theory we will discuss in this chapter represents a refinement of the CNT which recognizes this difference. It is often referred to in literature as two-step nucleation theory (2-SNT)[5–7]; its development took place mainly during the last three decades and was fueled by considerable progress in measurement devices allowing for the observation of ever smaller entities in solution[8–10]. We will first introduce the two theories on a conceptual level before putting them on a more rigorous theoretical footing. A schematic illustration of the two theories is shown in Figure 2.1 where different key states of the solution and of the newly forming phase are depicted. We depict these states in relation to the number of molecules that are contained in the new phase and the number of molecules with crystalline order in this phase. In both theories the initial state for primary homogeneous nucleation is a clear, supersaturated solution (S > 1) containing a number of solute molecules that exhibit no crystalline order (top left corner in Figure 2.1) and a final state consisting of one or more macroscopic crystals (bottom right corner). However, the pathways connecting the two states are rationalized in different ways in the CNT (blue arrows) and 3 CHAPTER 2. NUCLEATION the 2-SNT (orange arrows); key states along the two pathways are discussed in the following. Classical nucleation theory states that crystalline clusters are forming from the supersaturated solution through simultaneous fluctuations in density and order. Building blocks of crystals (assumed here to be solute molecules) can attach to or detach from a cluster in a step-by-step fashion. When molecules are attaching/detaching, the blue pathway laid out in Figure 2.1 is traveled reversibly depending on the rates of molecule attachment and detachment. We will show in Section 2.2.1 that the attachment of molecules to a cluster is energetically unfavorable until a critical number of molecules is reached while it is favored beyond this number. Two-step nucleation theory, on the other hand, treats the evolution of density and structure of the newly formed phase independently from each other. Namely, the 2-SNT postulates that density fluctuations first lead to the formation of disordered, liquid-like clusters (or droplets), which exhibit a higher density than the original clear liquid. Only after this step, crystalline order is achieved through rearrangement of the molecules within that cluster. 4 2.2. Homogeneous Nucleation number of molecules in new phase(s) molecules in solution free molecules and disordered, liquid‐like cluster order free molecules and free molecules and crystalline crystalline cluster cluster in liquid‐like cluster crystalline ... with molecules of free molecules and free molecules and crystalline nucleus crystalline nucleus inside disordered cluster number macroscopic crystal Figure 2.1: Conceptual picture of the mechanisms of nucleation according to classical nucleation theory (CNT; blue arrows) and to two step nucle- ation theory (2-SNT; orange arrows). Both theories consider a supersaturated solution as their starting point. According to both theories, clusters are form- ing/disintegrating through the attachment/detachment of building units. In the CNT, clusters are assumed to exhibit the final crystal structure immediately when building units attach. In contrast, the 2-SNT assumes that nucleation proceeds through the formation of disordered, liquid-like clusters in a first step, while the formation of structured clusters occurs from these droplets in a second step. Upon reaching a critical cluster size – the so-called nucleus size – the attachment of further building units is energetically favored; ultimately leading to the formation of a macroscopic crystal. 5 CHAPTER 2. NUCLEATION 2.2.1 Classical Nucleation Theory Classical nucleation theory dates back to the work of Gibbs, Becker and Döring, Volmer and many others [11, 12]. The interested reader can find the full derivation, historical details, and various technical aspects of CNT in the classical works of Kashchiev and deBenedetti [1, 13]. In order to describe the thermodynamic aspects of CNT, we derive the energy difference between a solution and a solution containing a cluster of n molecules. As hinted at in the introduction, the total change in the system free energy, ∆Gn, that is, the work of cluster formation, is given by the sum of two terms: an energy gain due to formation of a crystalline bulk phase and an energy loss caused by the formation of an interface between the new and the parent phase.
Details
-
File Typepdf
-
Upload Time-
-
Content LanguagesEnglish
-
Upload UserAnonymous/Not logged-in
-
File Pages30 Page
-
File Size-