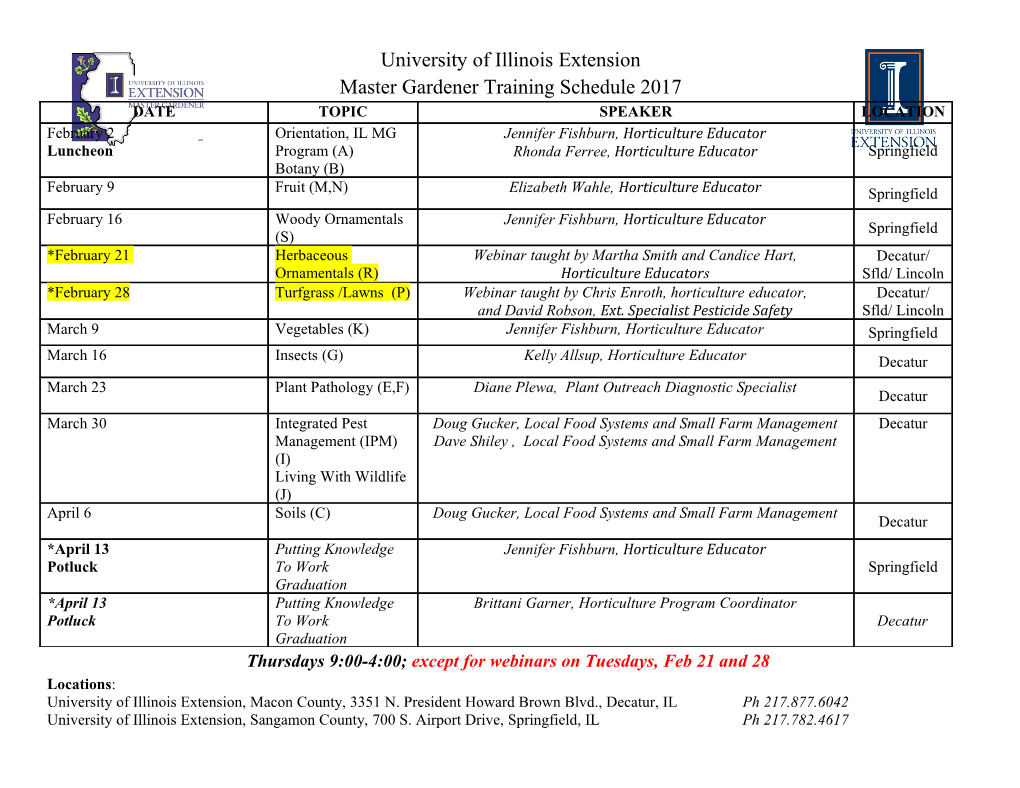
Heriot-Watt University 1 Design and Performance of an InGaAs–InP Single-Photon Avalanche Diode Detector Sara Pellegrini, Ryan E. Warburton, Lionel J. J. Tan, Jo Shien Ng, Andrey B. Krysa, Kristian Groom, John P. R. David, Sergio Cova, Michael J. Robertson, and Gerald S. Buller Abstract—This paper describes the design, fabrication, and performance of planar-geometry InGaAs–InP devices which were II. DEVICE STRUCTURE specifically developed for single-photon detection at a wavelength of 1550 nm. General performance issues such as dark count rate, single-photon detection efficiency, afterpulsing, and jitter are The devices studied in this paper are of planar geometry and described. are based on the separate absorption, grading and multiplication region APDs [12] commonly used for I. INTRODUCTION applications requiring internal gain of weak input optical signals. In this structure the optical absorption is performed in INGLE-PHOTON counting and single-photon timing the In0.53Ga0.47 As, which is not suitable for multiplication, S have become increasingly important in a number of since tunnelling in this material [13] would take place at a applications such as time-resolved photoluminescence [1], lower electric field than that required for impact ionization. optical time-domain reflectometry (OTDR) [2] and time-of- Instead, multiplication takes place in the wider gap InP. In flight laser ranging [3] and imaging. More recently they have order to smooth the large valence band discontinuity between been employed in quantum key distribution [4] and InGaAs and InP (see below), a suitable quaternary grading noninvasive testing of VLSI circuits [5]. Commercially layer of InGaAsP is added between the two materials. available InGaAs-InP avalanche photodiodes (APDs) designed It is important to notice that the design criteria for the for use in linear multiplication mode have been tested in devices described in this paper are similar to linear Geiger mode [6], [7] in order to extend the spectral range of multiplication APDs except in three important respects. 1) The efficient single-photon detection to wavelengths greater than design must allow low temperature operation (at least down to that afforded by the currently available Si-based single-photon 150 K) in order to reduce the DCR due to thermally generated avalanche diode (SPAD) detectors (i.e., wavelengths greater carriers, therefore the difference between the punch-though than 1 m). These InGaAs–InP detectors have exhibited good voltage and the breakdown voltage must be of at least 30 V, single-photon detection efficiency (SPDE) (SPDE > 10%) and since the former remains almost unchanged, while the latter is subnanosecond timing jitter, however they have limited reduced with decreasing temperature. 2) The design must take counting rates due to the severe deleterious effects of the into account the higher electric fields used in comparison with afterpulsing phenomenon. Alternative approaches to photon- linear multiplication devices. In particular, it is necessary to counting have also been developed such as quantum-dot (QD) avoid as far as possible tunneling effects, even those with low field effect transistors [8] and QD resonant tunnelling diodes probability, since they will also increase the DCR. This is [9] which have shown promising results in terms of SPDE at achieved by having a low electric field in the InGaAs. 3) The wavelengths around 684 nm [10]. Superconducting single- mask design incorporates different diffused area curvatures in photon detectors [11] have demonstrated a SPDE of order to accommodate the smaller volume structures needed approximately 5% at a wavelength of 1.55 m when cooled to for low DCRs whilst avoiding edge breakdown effects, such as a temperature of 4 K. the 10-µm diameter devices (see later). The specific choice of This paper describes the design, fabrication and the zinc diffused planar geometry was based on previous characterization of planar geometry InGaAs–InP avalanche studies done by our research groups, which highlighted diode detectors, which have been specifically designed for consistently lower DCRs in devices fabricated using this single-photon detection. We describe the temperature approach [6], [7], [14]. dependence of the device SPDE, dark count rate (DCR), noise Fig. 1(a) is a schematic showing the principle of device equivalent power (NEP), and jitter, focusing on the effect of operation in relation to its energy band diagram. When a the critical InGaAsP grading layer between the narrow-gap photon is absorbed in the InGaAs layer, an electron–hole pair InGaAs absorption layer and the wide-gap InP multiplication is generated. The electron is swept toward the back contact, layer. This study represents a fabrication program for planar while the hole drifts in the depleted InGaAs layer toward the InGaAs–InP SPADs and highlights some important issues in InP multiplication region where it undergoes impact device design. ionization. The intermediate bandgap InGaAsP is introduced between InGaAs and InP to ensure efficient transfer of holes across the large valence band discontinuity. In this paper we Heriot-Watt University 2 Fig. 2. Microscope image of a 40-_m-diameter InGaAs–InP SPAD. the curvature at the edge of the device, thus reducing the likelihood of edge breakdown [17], where the device will preferentially exhibit avalanche breakdown at the edge of the active area rather than in the central region, hence significantly lowering the SPDE. The likelihood of edge breakdown can be further reduced by the introduction of floating guard rings (FGR) [12] that decrease the electric field at the edges of the main junction. Fig. 1. (a) Energy band diagram of the device under reverse bias conditions. An optical microscope image of the device prior to bonding (b) Schematic cross section of a planar SPAD with one floating guard ring, a top p-contact to the active area, and a bottom n-contact to the substrate. is shown in Fig. 2. The active area of the device is defined by the opening for the deeper zinc diffusion, and the metal contact. Both the central diffusions and the floating guard rings are concentric. The contact is formed by evaporated will show the effect of the grading layer composition on the Au-Zn-Au for the top p-side, and InGe–Au for the n-substrate. SPDE of the InGaAs–InP SPAD. The device material was grown epitaxially by metal organic III. DEVICE CHARACTERISTICS chemical vapor deposition. As shown in Fig. 1(b), the device The reverse current–voltage characteristics showed a similar consists of a 2.5-µm-thick layer of lightly n-doped InGaAs for behavior for both structures. The only evident difference in the efficient absorption of photons at a wavelength of 1.55 µm, curves shown in Fig. 3 for the dark current measured in 10- m- and a 1- m-thick layer of nominally undoped InP for diameter devices from both structures at room temperature is multiplication to take place. The charge sheet layer is a 300- the position of the punch-through voltage (V ). This is the nm-thick InP n-doped at 6 × 1016 cm-3, which is needed to PT voltage at which the depletion region extends into the In-GaAs reduce the electric field from the InP multiplication region to layer and efficient collection of the carriers generated by the InGaAs absorption layer. For the grading layer, two main absorption of 1.55-µm wavelength light can take place. The device designs were grown: 1) with a graded region consisting dark current (I ) and breakdown voltages (V ) are the same of one quaternary with an exactly intermediate bandgap D BD for both structures. The dark current at room temperature was between InGaAs and InP, which we shall call SPAD-1Q and measured to be 3 nA at 95% of V , and V varied between 2) with a graded region composed of three sublayers of BD BD 105 and 110 V across approximately 10 mm of wafer. The stepped bandgap, which we shall call SPAD-3Q. Our previous difference in the punch-through voltage value is most likely attempts to utilize a continuously varying gap were not due to the slight thickness variation between the two structures successful. The p-n junction is fabricated by diffusing the p- in the top InP layer. From Fig. 4, it can also be noticed that the type dopant zinc into the top InP region. In order to minimize punch-through voltage does not change significantly with the DCR of the device, a planar geometry device was formed temperature, as expected, nor does the value of the using the diffusion of the p-type Zn dopant alone. The use of photocurrent measured just above punch-through with an etch and regrowth techniques [15], [16] was avoided in order incident light of 1.55-µm wavelength. The breakdown voltage to reduce interface states that may contribute to dark count and decreases linearly with temperature with a coefficient of ~0.17 afterpulse levels. The active area is shaped by the diffusion of V/K, which is consistent with previously published results [7]. zinc via two separate processes using different mask sets as For the characterization of these detectors in terms of first suggested by Liu et al. [12]. This double diffusion reduces photon-counting performance, we used the setup shown in Heriot-Watt University 3 Fig. 3. Reverse dark current measured in a selection of 10-_m-diameter devices from both structures SPAD-1Q and SPAD-3Q at room temperature. Fig. 5. Experimental setup for photon-counting characterization of the InGaAs–InP SPAD. MB corresponds to the microscope baseplate, and BS is a pellicle beamsplitter. Fig. 5. Two lasers were used for the characterization: a precision alignment and focusing on the detector. White light passively Q-switched diode laser [18] for the timing and an infrared camera were used to image the devices.
Details
-
File Typepdf
-
Upload Time-
-
Content LanguagesEnglish
-
Upload UserAnonymous/Not logged-in
-
File Pages7 Page
-
File Size-