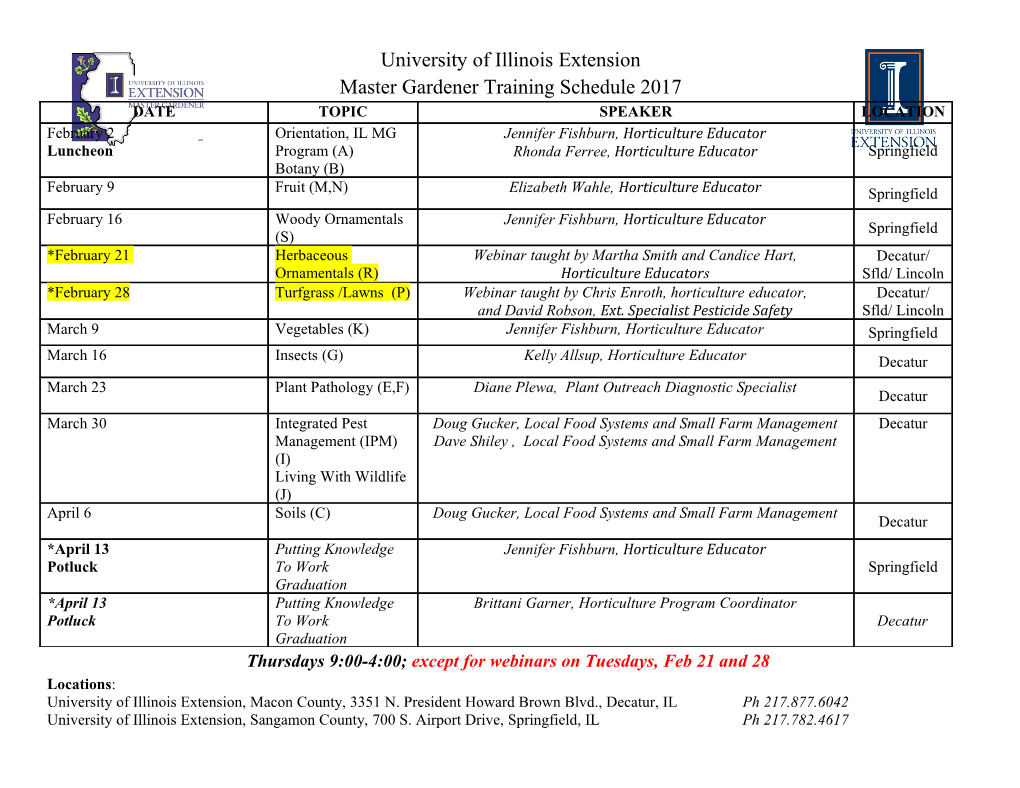
Cryogenics for superconducting devices Philippe Lebrun Director, Joint Universities Accelerator School JUAS 2019 Course 2 19 February 2019 Contents • Introduction • Cryogenic fluids • Heat transfer & thermal insulation • Thermal screening with cold vapour • Refrigeration & liquefaction • Thermometry Ph. Lebrun JUAS 2019 Cryogenics for SC devices 2 • 훋훠훖훐훓, 훐훖훓 το 1 deep cold [Arist. Meteor.] 2 shiver of fear [Aeschyl. Eumenid.] • cryogenics, that branch of physics which deals with the production of very low temperatures and their effects on matter Oxford English Dictionary 2nd edition, Oxford University Press (1989) • cryogenics, the science and technology of temperatures below 120 K New International Dictionary of Refrigeration 4th edition, IIF-IIR Paris (2015) Ph. Lebrun JUAS 2019 Cryogenics for SC devices 3 Characteristic temperatures of cryogens Normal boiling Critical point Cryogen Triple point [K] point [K] [K] Methane 90.7 111.6 190.5 Oxygen 54.4 90.2 154.6 Argon 83.8 87.3 150.9 Nitrogen 63.1 77.3 126.2 Neon 24.6 27.1 44.4 Hydrogen 13.8 20.4 33.2 Helium 2.2 (*) 4.2 5.2 (*): l Point Ph. Lebrun JUAS 2019 Cryogenics for SC devices 4 Useful range of liquid cryogens & critical temperature of superconductors Nb3Sn Nb-Ti Mg B2 YBCO Bi-2223 Helium Below Patm Above Patm Hydrogen Neon Nitrogen Argon Oxygen 0 20 40 60 80 100 120 140 160 180 T [K] Ph. Lebrun JUAS 2019 Cryogenics for SC devices 5 Cooling of superconducting devices LHe 1.9 K LIN ~70 K SCHe 4.5 K LHe 4.2 K Ph. Lebrun JUAS 2019 Cryogenics for SC devices 6 Contents • Introduction • Cryogenic fluids • Heat transfer & thermal insulation • Thermal screening with cold vapour • Refrigeration & liquefaction • Thermometry Ph. Lebrun JUAS 2019 Cryogenics for SC devices 7 Properties of cryogens compared to water Property He N2 H2O Normal boiling point [K] 4.2 77 373 Critical temperature [K] 5.2 126 647 Critical pressure [bar] 2.3 34 221 Liq./Vap. density (*) 7.4 175 1600 Heat of vaporization (*) [J.g-1] 20.4 199 2260 Liquid viscosity (*) [mPl] 3.3 152 278 (*) at normal boiling point Ph. Lebrun JUAS 2019 Cryogenics for SC devices 8 Specific heat of helium and materials Ph. Lebrun JUAS 2019 Cryogenics for SC devices 9 Specific heat of helium and materials Ph. Lebrun JUAS 2019 Cryogenics for SC devices 10 Vaporization of normal boiling cryogens under 1 W applied heat load Let ℎ be the enthalpy of the fluid At constant pressure 푄ሶ = 퐿푣푚ሶ with 퐿푣 = ℎ푣푎푝 − ℎ푙푞 [l.min-1] Cryogen [mg.s-1] [l.h-1] (liquid) (gas NTP) Helium 48 1.38 16.4 Nitrogen 5 0.02 0.24 Ph. Lebrun JUAS 2019 Cryogenics for SC devices 11 Amount of cryogens required to cool down 1 kg iron Assuming perfect heat exchange between iron and the fluid 푇푛푡푎푙 푓푛푎푙 푠푎푡 න 푀퐹푒퐶퐹푒푑푇 = 푚 퐿푣 + ℎ푣푎푝 − ℎ푣푎푝 ≈ 푚 퐿푣 + 퐶푝 푇푓푛푎푙 − 푇푠푎푡 푇푓푛푎푙 Latent heat and Using Latent heat only enthalpy of gas LHe from 290 to 4.2 K 29.5 litre 0.75 litre LHe from 77 to 4.2 K 1.46 litre 0.12 litre LN2 from 290 to 77 K 0.45 litre 0.29 litre Ph. Lebrun JUAS 2019 Cryogenics for SC devices 12 Phase diagram of helium 10000 SOLID 1000 SUPER- l LINE CRITICAL He II He I CRITICAL 100 POINT PRESSURIZED He II SATURATED He I (Subcooled liquid) Pressure[kPa] 10 VAPOUR SATURATED He II 1 0 1 2 3 4 5 6 Temperature [K] Ph. Lebrun JUAS 2019 Cryogenics for SC devices 13 Helium as a cooling fluid Phase domain Advantages Drawbacks Fixed temperature Two-phase flow Saturated He I High heat transfer Boiling crisis Supercritical Monophase Non-isothermal Negative J-T effect Density wave instability Low temperature Second-law cost He II High conductivity Subatmospheric Low viscosity Ph. Lebrun JUAS 2019 Cryogenics for SC devices 14 Contents • Introduction • Cryogenic fluids • Heat transfer & thermal insulation • Thermal screening with cold vapour • Refrigeration & liquefaction • Thermometry Ph. Lebrun JUAS 2019 Cryogenics for SC devices 15 Typical heat transfer coefficients at cryogenic temperatures • Same basic processes as at temperatures above ambiant, but large variations in - absolute values - dependence on temperature • These variations can be exploited for - cooling equipment - thermal insulation of cryostats • Particular importance of two- phase heat transfer Ph. Lebrun JUAS 2019 Cryogenics for SC devices 16 Non-linear heat transfer to liquid cryogens Pool boiling nitrogen ~ 1.5 105 W/m2 Ph. Lebrun JUAS 2019 Cryogenics for SC devices 17 Non-linear heat transfer to liquid cryogens Pool boiling helium 104 W/m2 Ph. Lebrun JUAS 2019 Cryogenics for SC devices 18 Heat conduction in solids 푑푇 SA • Fourier’s law 푄ሶ = 푘 푇 퐴 T 2 푐표푛푑 푑푥 • Thermal conductivity 푘 푇 [W/m.K] Q c o n dx 퐴 푇2 Integral form 푄ሶ푐표푛푑 = 푘 푇 푑푇 • dT 퐿 푇1 푇 [Thermal conductivity integral 2 푘 푇 푑푇 [W/m • 푇1 • Thermal conductivity integrals for standard construction materials are tabulated T 1 Ph. Lebrun JUAS 2019 Cryogenics for SC devices 19 Thermal conductivity integrals of selected materials [W/m] From vanishingly low temperature 20 K 80 K 290 K up to OFHC copper 11000 60600 152000 DHP copper 395 5890 46100 1100 aluminium 2740 23300 72100 2024 aluminium alloy 160 2420 22900 AISI 304 stainless steel 16.3 349 3060 G-10 glass-epoxy composite 2 18 153 Ph. Lebrun JUAS 2019 Cryogenics for SC devices 20 Non-metallic composite support post with heat intercepts 5 K cooling line (SC He) Aluminium intercept plates glued to G-10 column Aluminium strips to thermal shield at 50-75 K Ph. Lebrun JUAS 2019 Cryogenics for SC devices 21 Thermal radiation • Wien’s law – Maximum of black-body power spectrum 휆푚푎푥 푇 = 2898 [μm. K] • Stefan-Boltzmann’s law 4 – Black body 푄ሶ푟푎푑 = 퐴 푇 with = 5.67 10−12 W/m2K4 4 – «Gray» body 푄ሶ푟푎푑 = 휀 퐴 푇 with 휀 surface emissivity Qrad1 T T > T – Between «gray» surfaces at temperatures 푇1 and 푇2 1 2 1 ሶ 4 4 푄푟푎푑 = 퐸 퐴 (푇2 − 푇1 ) 1 Qrad2 2 with 퐸 function of 휀1, 휀2 and geometry of facing surfaces Ph. Lebrun JUAS 2019 Cryogenics for SC devices 22 Emissivity of technical materials at low temperatures Radiation from 290 K Radiation from 77 K Surface at 77 K Surface at 4.2 K Stainless steel, as found 0.34 0.12 Stainless steel, mech. polished 0.12 0.07 Stainless steel, electropolished 0.10 0.07 Stainless steel + Al foil 0.05 0.01 Aluminium, as found 0.12 0.07 Aluminium, mech. polished 0.10 0.06 Aluminium, electropolished 0.08 0.04 Copper, as found 0.12 0.06 Copper, mech. Polished 0.06 0.02 Ph. Lebrun JUAS 2019 Cryogenics for SC devices 23 Residual gas conduction • Two different regimes, depending upon the relative values of heat transfer distance 푑 and mean free path of gas molecules 휆푚표푙푒푐푢푙푒 • Viscous regime – At higher pressure 휆푚표푙푒푐푢푙푒 ≪ 푑 푑푇 – Classical conduction 푄ሶ = 퐴 푘 푇 푟푒푠푑푢푎푙 푑푥 T1 T2 – Thermal conductivity 푘(푇) independant of pressure • Molecular regime d – At lower pressure 휆푚표푙푒푐푢푙푒 ≫ 푑 – Kennard’s law 푄ሶ푟푒푠푑푢푎푙 = 퐴 훼 푇 Ω 푃 (푇2 − 푇1) – Heat transfer proportional to pressure, independant of spacing between surfaces – Ω depends on gas species – Accommodation coefficient 훼 푇 depends on gas species, 푇1, 푇2 and geometry of facing surfaces Ph. Lebrun JUAS 2019 Cryogenics for SC devices 24 Multi-layer insulation (MLI) • Complex system involving three heat transfer processes – 푄ሶ푀퐿퐼 = 푄ሶ푟푎푑 + 푄ሶ푐표푛푡푎푐푡 + 푄ሶ푟푒푠푑푢푎푙 – With 푛 reflective layers of equal emissivity, 푄ሶ푟푎푑~ 1Τ(푛 + 1) – Due to parasitic contacts between layers, 푄ሶ푐표푛푡푎푐푡 increases with layer density – 푄ሶ푟푒푠푑푢푎푙 due to residual gas trapped between layers, scales as 1Τ푛 in molecular regime – Non-linear behaviour requires layer-to-layer modeling • In practice – Typical data available from (abundant) literature – Measure performance on test samples Ph. Lebrun JUAS 2019 Cryogenics for SC devices 25 Typical heat fluxes at vanishingly low temperature between flat plates [W/m2] Black-body radiation from 290 K 401 Black-body radiation from 80 K 2.3 Gas conduction (100 mPa He) from 290 K 19 Gas conduction (1 mPa He) from 290 K 0.19 Gas conduction (100 mPa He) from 80 K 6.8 Gas conduction (1 mPa He) from 80 K 0.07 MLI (30 layers) from 290 K, pressure below 1 mPa 1-1.5 MLI (10 layers) from 80 K, pressure below 1 mPa 0.05 MLI (10 layers) from 80 K, pressure 100 mPa 1-2 Ph. Lebrun JUAS 2019 Cryogenics for SC devices 26 Cross-section of LHC dipole cryostat Ph. Lebrun JUAS 2019 Cryogenics for SC devices 27 LHC cryostat heat inleaks at 1.9 K Measured 푄ሶ = 푚ሶ ∆ℎ(푃, 푇) He property tables LHC sector (2.8 km) Total S7-8 @ 1.9 K 600 500 400 On full LHC cold sector (2.8 km) 300 [W] - Measured 560 W, i.e. 0.2 W/m 200 - Calculated 590 W, i.e 0.21 W/m 100 0 Calc. Meas. Ph. Lebrun JUAS 2019 Cryogenics for SC devices 28 Contents • Introduction • Cryogenic fluids • Heat transfer & thermal insulation • Thermal screening with cold vapour • Refrigeration & liquefaction • Thermometry Ph. Lebrun JUAS 2019 Cryogenics for SC devices 29 Vapour cooling of cryostat necks and supports with perfect heat transfer Cross-section A • Assuming perfect heat transfer between solid and vapour, i.e. 푇푠표푙푑(푥) = 푇푣푎푝표푟(푥) = 푇(푥) . m vapour flow Cp(T) 푄ሶ푐표푛푑 = 푄ሶ푏푎푡ℎ + 푚ሶ 퐶푝 푇 (푇 − 푇푏푎푡ℎ) 푑푇 퐴 푘 푇 = 푄ሶ + 푚ሶ 퐶 푇 (푇 − 푇 ) T T 푑푥 푏푎푡ℎ 푝 푏푎푡ℎ x Qcon • 퐶푝(푇) specific heat of vapour • 푘 푇 thermal conductivity of support • 푄ሶ푏푎푡ℎ can be calculated by numerical integration for Tbath – different cryogens Qbath LHe – different values of aspect ratio 퐿Τ퐴 – different values of vapour flow Ph.
Details
-
File Typepdf
-
Upload Time-
-
Content LanguagesEnglish
-
Upload UserAnonymous/Not logged-in
-
File Pages74 Page
-
File Size-