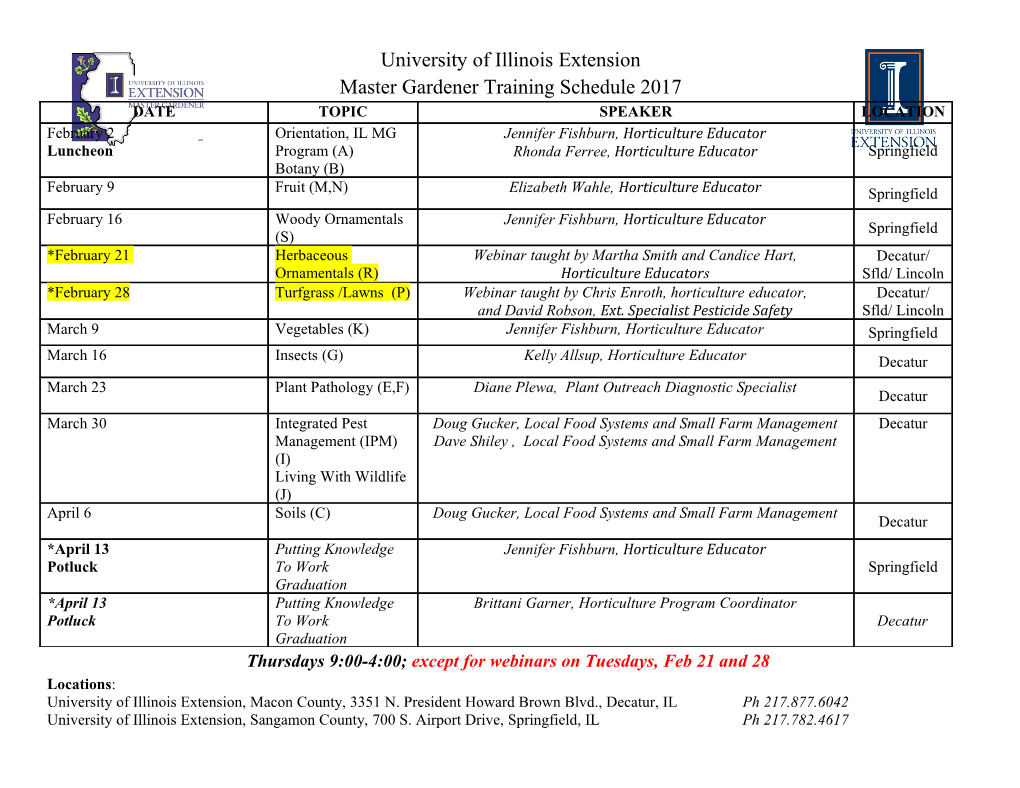
Planetary and Space Science 145 (2017) 71–77 Contents lists available at ScienceDirect Planetary and Space Science journal homepage: www.elsevier.com/locate/pss Shape of boulders ejected from small lunar impact craters Yuan Li a,*, A.T. Basilevsky b, Minggang Xie a, Wing-Huen Ip a,c a Space Science Institute, Macau University of Science and Technology, Macau b Vernadsky Institute of Geochemistry and Analytical Chemistry, Russian Academy of Sciences, Moscow 119991, Russia c Institute of Astronomy, National Central University, 32054 Chung-Li, Taiwan ARTICLE INFO ABSTRACT Keywords: The shape of ejecta boulders from 7 lunar impact craters <1 km in diameter of known absolute age was measured Moon to explore whether it correlates with the crater age and the boulder size. The boulders were mapped and then Shape of boulders measured by rectangular fitting and the shape was represented by the axial ratio or aspect ratio (A) of the Axial ratio rectangle. The main conclusions from the analysis of our measurement results are: 1) the percentages of the Crater age number of boulders of studied craters decrease with the increase of the axial ratio. Most (~90%) of the boulders have the axial ratio in the range of 1–2; no boulder with axial ratio larger than 4 was found. 2) the axial ratios of mare ejecta boulders decrease with their exposure time, whereas that for highland ones show unchanged trend. This difference may be probably due to target properties. 3) The shape of ejecta boulders are influenced by mechanical strength of bedrocks and space erosion. 4) surface peak stresses caused by thermal fatigue maybe play a significant erosion role in the shape of boulders of various diameter. 1. Introduction micrometeoroid and meteoroid bombardment and thermal stresses due to diurnal temperature changes (e.g., Clark et al., 2002). Solar wind, Impact craters and rocks/boulders are the predominant features on cosmic ray and electromagnetic radiation change the optical properties the lunar surface. In most cases, rocks appear on the lunar surface as a of the exposed materials within the rather thin surface layer. Microme- result of ejections from impact craters and come from the regolith layer teoroid bombardment was found to work mostly in the form of and bedrock basement underlying the regolith. The bedrocks in lunar sand-blasting without destroying the rocks (Horz€ et al., 1975, 1977; maria are composed of various basalts. In highlands these are impact McDonnell, 1977). Only meteoroid bombardment and thermal stresses breccias, which can be essentially fragmental breccias or contain are considered as the major factors for the destruction of boulders on the different contents of solidified shock melt (e.g., Florensky et al., 1981; airless body surface (see e.g., Basilevsky et al., 2013, 2015; Cintala and Heiken et al., 1991). Accordingly, lunar rocks/boulders studied by us are Horz,€ 2008; Delbo et al., 2014; Horz€ et al., 1975; Molaro et al., 2016). fragments of basalts or impact breccias. It should be noted that catastrophic rupture of exposed rocks on the The shape of ejecta boulders may provide an insight into the impact airless body by meteoroid bombardment has a stochastic character with fragmentation process (e.g., Melosh, 1989; Senthil Kumar et al., 2014). some boulders destroyed very soon after their appearance on the surface Krishna and Kumar (2016) described shapes of boulders by rectangular while some other that may stay untouched for very long time (e.g., Horz€ fitting, and defined the axial ratio (or aspect ratio) between long and et al., 1975). Meanwhile the destruction process by thermal stress should short axes of rectangular as a measure of the boulder shape. They sug- be more uniform in time if given the same mass and similar gested that the axial ratio (or aspect ratio) of boulders depends on ejec- thermo-mechanical properties, as all sun-illuminated rocks are univer- tion velocity, and an increase in the ejection velocity leads to a decrease sally subjected to the same process. of the axial ratio for boulders. In the following, the axial ratio or aspect Generally, the larger rocks demand the higher impact energy to be ratio will be abbreviated as A. destroyed, thus the longer survival time could be expected for them (e.g., After their formation, boulders exposed on the airless body surface Horz€ et al., 1975). However the larger rocks are mechanically weaker due are affected by a number of agents: solar wind ion implantation and to the increased number and size of intrinsic flaws (Housen and Hol- sputtering, cosmic-ray bombardment, electromagnetic radiation, sapple, 1999). Moreover, the larger rock has the higher probability to be * Corresponding author. E-mail address: [email protected] (Y. Li). http://dx.doi.org/10.1016/j.pss.2017.07.014 Received 8 June 2017; Accepted 21 July 2017 Available online 26 July 2017 0032-0633/© 2017 The Authors. Published by Elsevier Ltd. This is an open access article under the CC BY license (http://creativecommons.org/licenses/by/4.0/). Y. Li et al. Planetary and Space Science 145 (2017) 71–77 Table 1 The list of location, terrain type, size and absolute age of the craters studied in this work. Crater Location Terrain Crater Crater Age name (Lat., Lon.) type size age determination (m) (Ma) technique and references South Ray À9.15,15.38 Highland 680 ~2 Radiometric (1,2) Unnamed 12.25, 62.24 Mare 200 5–10 Morphologic (3) A Unnamed À3.61,336.51 Mare 400 20–30 Morphologic (4) B Cone À3.63,342.57 Highland 340 ~26 Radiometric (5) North Ray À8.80,15.49 Highland 950 ~50 Radiometric (1,6) Unnamed À3.02,336.58 Mare 560 70 ± 30 Morphologic (7) D Camelot 20.21,30.73 Mare 650 ~100 Radiometric (7) (1) - Arvidson et al. (1975), (2) - Eugster (1999), (3) - Basilevsky and Head (2012), (4) - Basilevsky (1976), (5) - Turner et al. (1971), (6) - Borchardt et al. (1986), (7) - Li et al. (2017), (8) - Kirsten et al. (1973). Fig. 1. This image gives an illustration for the nominal diameter and A of identified boulders which located at the part of study area of North Ray crater. The boulder Table 2 encompassed by rectangle with width of ~5 m and length of ~6.5 m, has A of 1.3 and The basic information on images selected for the boulder counting for each of study craters. nominal diameter of ~5.7 m. Crater Image Id Pixel scale Local solar time Incidence name (m/pixel) (24-h clock) angle boulders with exposure time on the lunar surface. (degree) South Ray M119754107RE 0.45 15.70 56.15 2. Previous studies on the rock/boulder shape Unnamed M119449091RE 0.48 15.97 60.22 A The observed rocks can be represented as cuboid-shaped objects Unnamed M120005333LE 0.48 15.46 52.09 having three mutually perpendicular axes: the long axis a, medium axis b, B M144775952RE 0.44 8.64 50.39 Cone M168319885RE 0.29 14.49 37.40 and short axis c. It is obvious that in most cases a rock ejected to the lunar M175388134LE 0.28 9.01 44.87 surface should lie with its short axis upward. So, when we observe rocks Unnamed M142495666RE 0.51 10.45 44.88 from above, as in the case of the present study, most probably we see the C M166072850LE 0.49 16.14 69.03 long a and medium b dimensions of it (e.g., Demidov and Basilev- North Ray M144524996LE 0.45 8.88 47.18 Borya M127159138LE 0.40 9.95 46.42 sky, 2014). M135418902RE 0.51 15.81 65.29 Fujiwara et al. (1978) reported an impact experiment in which ~5 cm Unnamed M104662862LE 1.09 15.29 49.37 basalt blocks were shot with polycarbonate cylinders 0.8 cm in diameter D M1121393925E 0.98 9.13 43.13 with masses of 0.37 g at a velocity of 1–4 km/s. Statistical processing of Camelot M134991788LE 0.48 16.17 64.60 the geometry of the fragments yielded average values of b/a ¼ 0.73, and M162107606LE 0.43 7.14 73.85 ¼ Spook M144524996LE 0.45 8.89 47.18 c/a 0.5. Capaccioni et al. (1984) used a 1 g aluminum projectile shot at a velocity of ~10 km/s at triaxial ellipsoids with dimensions 30 Â 21 Â 15 cm and composed of specific concrete and obtained values impacted due to their larger area. The interplay of these factors are not of b/a ¼ 0.72 and c/a ¼ 0.49 which are similar to the result of Fujiwara well understood by now. Therefore, the issue of how meteoroid impacts et al. (1978). If to consider the a/b ratio (A), these experiments showed affect the survival times of boulders of various sizes demands addi- the average value to be ~1.4. tional studies. Demidov and Basilevsky (2014), as part of their study, measured 40 By modeling boulder/rock response to diurnal thermal forcing, relatively large boulders in six rocky areas of lunar surface using Lunar Molaro et al. (2017) concluded that lunar exposed boulders have weak Reconnaissance Orbiter Camera (LROC) Narrow Angle Camera (NAC) outer layer that is susceptible to surface disaggregation, so this disag- images and found that b/a ratio is 0.78 for highland rocks with a standard gregation should be the primary mechanism for thermal breakdown, deviation of 0.16 and 0.83 for mare rocks with a standard deviation of while the formation of large cracks that splits a boulder would 0.18. The Welch test showed that the distributions of the b/a parameters be secondary.
Details
-
File Typepdf
-
Upload Time-
-
Content LanguagesEnglish
-
Upload UserAnonymous/Not logged-in
-
File Pages7 Page
-
File Size-