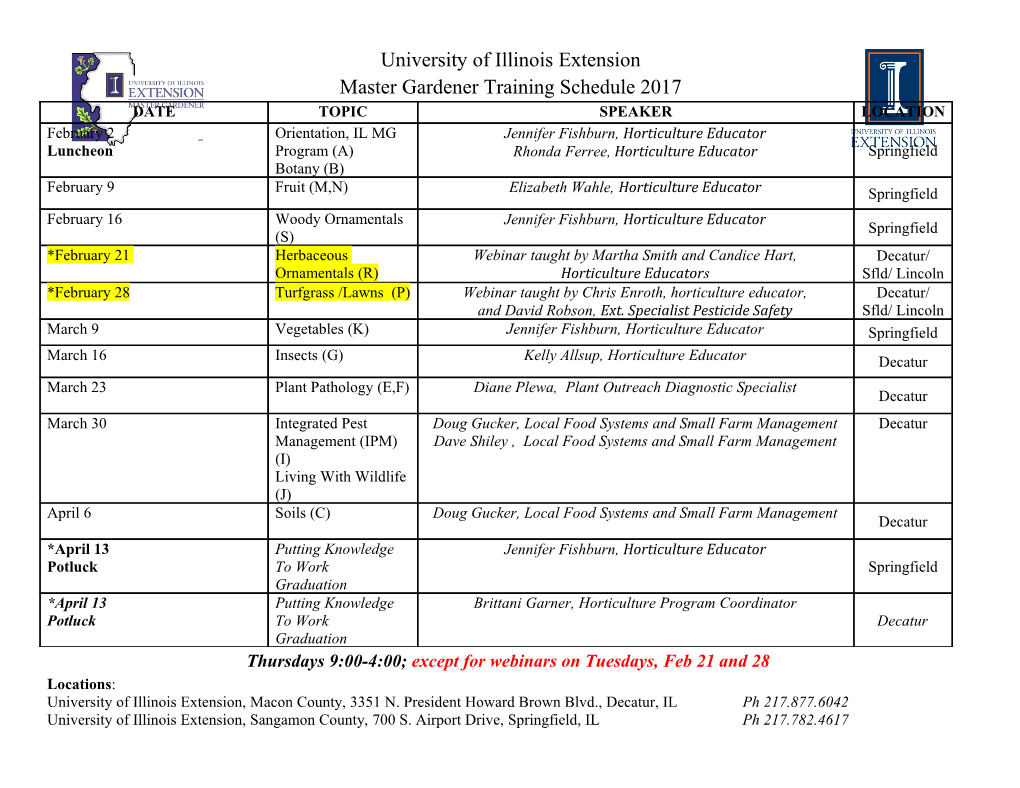
The p53-cathepsin axis cooperates with ROS to activate programmed necrotic death upon DNA damage Ho-Chou Tua,1, Decheng Rena,1, Gary X. Wanga, David Y. Chena, Todd D. Westergarda, Hyungjin Kima, Satoru Sasagawaa, James J.-D. Hsieha,b, and Emily H.-Y. Chenga,b,c,2 aDepartment of Medicine, Molecular Oncology, bSiteman Cancer Center, and cDepartment of Pathology and Immunology, Washington University School of Medicine, St. Louis, MO 63110 Edited by Stuart A. Kornfeld, Washington University School of Medicine, St. Louis, MO, and approved November 25, 2008 (received for review August 19, 2008) Three forms of cell death have been described: apoptosis, autophagic cells that are deprived of the apoptotic gateway to mediate cyto- cell death, and necrosis. Although genetic and biochemical studies chrome c release for caspase activation (Fig. S1) (9–11, 19, 20). have formulated a detailed blueprint concerning the apoptotic net- Despite the lack of caspase activation (20), DKO cells eventually work, necrosis is generally perceived as a passive cellular demise succumb to various death signals manifesting a much slower death resulted from unmanageable physical damages. Here, we conclude an kinetics compared with wild-type cells (Fig. 1A, Fig. S2, and data active de novo genetic program underlying DNA damage-induced not shown). To investigate the mechanism(s) underlying BAX/ necrosis, thus assigning necrotic cell death as a form of ‘‘programmed BAK-independent cell death, we first examined the morphological cell death.’’ Cells deficient of the essential mitochondrial apoptotic features of the dying DKO cells. Electron microscopy uncovered effectors, BAX and BAK, ultimately succumbed to DNA damage, signature characteristics of necrosis in DKO cells after DNA exhibiting signature necrotic characteristics. Importantly, this geno- damage, including the loss of plasma membrane integrity, the toxic stress-triggered necrosis was abrogated when either transcrip- spillage of intracellular contents, the swelling of organelles, the tion or translation was inhibited. We pinpointed the p53-cathepsin appearance of translucent cytosolic compartment, and the forma- axis as the quintessential framework underlying necrotic cell death. tion of intracellular vacuoles (Fig. 1B). We next determined one of p53 induces cathepsin Q that cooperates with reactive oxygen species the biochemical hallmarks of necrosis-the release of high mobility (ROS) to execute necrosis. Moreover, we presented the in vivo group box I (HMGB1) protein, a proinflammatory cytokine (21). evidence of p53-activated necrosis in tumor allografts. Current study lays the foundation for future experimental and therapeutic discov- HMGB1 functions in the nucleus as an architectural chromatin- eries aimed at ‘‘programmed necrotic death.’’ binding factor that bends DNA, stabilizes nucleosomes, and facil- itates transcription, whereas outside the cell it is a potent mediator necrosis ͉ BAX ͉ BAK ͉ apoptosis ͉ caspase-independent cell death of inflammation, cell migration and metastasis (22). Indeed, HMGB1 was released into the culture medium when DKO cells died upon DNA insults (Fig. 1C). Analyses revealed a 2-step release ells constantly interpret environmental cues before making a of HMGB1-it first translocated from the nucleus to the cytosol Ϸ8 correct life versus death decision to assure proper operation of C h after genotoxic stress (Fig. 1D), followed by the diffusion to the all biological processes (1). Impaired or overactive death results in extracellular milieu once the plasma membrane integrity was lost human illness including cancer, autoimmune disorders, neurode- C generative diseases, stroke, and myocardial infarction (2). Research (Fig. 1 ). Double staining of necrotic cells with Annexin-V and PI focused on the cell death/survival control provides a vantage point have been shown in dsRNA- and TNF-induced necrosis (ref. 23 and T. Vanden Berghe and P. Vandenabeele, personal communica- for the development of therapeutic agents that specifically trigger CELL BIOLOGY or prevent cell death (3, 4). Based on the morphological features, tions). By analogy, the dying Bax and Bak DKO cells were stained 3 forms of cell death have been described: apoptosis, autophagic cell positively with both Annexin-V and propidium iodide (Fig. S2). death, and necrosis (5–7). Among the 3 distinct forms of cell death, Although some cells displayed increased double-membrane auto- apoptosis is best studied. The evolutionarily conserved signaling phagosomes and conversion of LC3-I to LC3-II was observed (Fig. cascade, consisting of the BCL-2 family, the adaptor protein Apaf-1, S3), knockdown of Beclin1 and ATG5 provided minimal protection and the caspase family, outlines the essential apoptotic network (8). at 3 days after DNA damage (Fig. S4). Furthermore, over- The ‘‘activator’’ BH3-only molecules, BID, BIM, and PUMA, expression of BCL-2 or BCL-XL had no effects, supporting that convey apoptotic signals to trigger homo-oligomerization of mul- DNA damage-induced death in DKO cells is not caused by mito- tidomain proapoptotic BAX and BAK, which in turn permeabilize chondrion-dependent apoptosis (Fig. S5A). Remarkably, DNA mitochondria, leading to the efflux of cytochrome c, the assembly damage-induced necrosis in DKO cells was completely blocked by of apoptosome, and the ultimate activation of caspases (9–11). either actinomycin D (ActD) or cycloheximide (CHX) that inhibits Autophagy serves as either a survival or a death mechanism transcription and translation, respectively (Fig. 2 A and B). More- depending upon the context of the signaling events (12). Although over, transient cycloheximide treatment enhanced the clonogenic necrosis is generally perceived as an unavoidable consequence of extreme physicochemical stress, recent studies have indicated a molecular control of tumor necrosis factor (TNF)/Fas and DNA Author contributions: H.-C.T., D.R., and E.H.-Y.C. designed research; H.-C.T., D.R., G.X.W., alkylation induced necrosis (13–18). However, whether necrosis D.Y.C., H.K., and S.S. performed research; H.-C.T., D.R., G.X.W., D.Y.C., T.D.W., J.J.-D.H., and triggered by other intrinsic death signals is similarly regulated and E.H.-Y.C. analyzed data; and E.H.-Y.C. wrote the paper. more importantly, whether necrotic cell death recruits de novo The authors declare no conflict of interest. genetic programs in analogy to apoptosis remain undetermined. This article is a PNAS Direct Submission. 1 H.-C.T. and D.R. contributed equally to this work. Results 2 To whom correspondence should be addressed. E-mail: [email protected]. DNA Damage Activates a de Novo Genetic Program to Execute This article contains supporting information online at www.pnas.org/cgi/content/full/ Necrotic Death in Bax and Bak DKO Cells. To interrogate nonapo- 0808173106/DCSupplemental. ptotic cell death, we used Bax and Bak double-knockout (DKO) © 2009 by The National Academy of Sciences of the USA www.pnas.org͞cgi͞doi͞10.1073͞pnas.0808173106 PNAS ͉ January 27, 2009 ͉ vol. 106 ͉ no. 4 ͉ 1093–1098 Downloaded by guest on September 26, 2021 p53 Transactivates Cathepsin Q to Trigger Necrotic Death in Bax and Bak DKO Cells. To pinpoint the responsible transcription factor(s) in orchestrating this necrotic program, we investigated several candi- dates known to control cell death and identified p53 as the key necrotic programmer. Overexpression of a dominant negative mutant of p53 or retrovirus-mediated stable knockdown of p53 protected DKO cells from DNA damage-induced necrosis (Fig. 2 D and E and Fig. S6). To identify the molecular signatures underlying the observed necrosis, we performed gene expressing profiling on DKO cells upon DNA damage and recognized up- regulation of several lysosomal cysteine proteases including cathep- sin F, H, and Q, but not cathepsin B and L (data not shown). The observed induction of cathepsin F, H, and Q was confirmed by quantitative RT-PCR analyses (Fig. 3A). Importantly, the up- regulation of these cathepsins was abrogated when p53 was knocked down, positioning p53 in orchestrating the cathepsin program (Fig. 3A). The importance of individual cathepsins in necrosis was investigated with shRNA-mediated stable knockdown of respective cathepsins (Fig. S7). Knockdown of cathepsin Q, but not F or H, protected DKO cells from DNA damage-induced necrosis acti- vated by etoposide (Fig. 3B). Furthermore, deficiency of cathepsin Q also protected DKO cells from UV- and camptothecin-induced necrosis (Fig. 3C and data not shown). Although cathepsin Q was barely detected by anti-cathepsin Q Western blot, DNA damage induced up-regulation of cathepsin Q protein that was readily detectable (Fig. S7D and S8A). Real time RT-PCR revealed a ubiquitous, mostly low-level expression of cathepsin Q in all mouse tissues except lung where no message was detected (Fig. S8B), which contrasts the prior reported placenta-restricted expression (24). Indeed, the expression of cathepsin Q protein could be detected in bone marrow (Fig. S8C). To directly link p53 to the transactivation of cathepsin Q, we searched for consensus p53 binding sites based on the p53MH algorithm (25) and identified one candidate p53-binding site located at the intron 1 of cathepsin Q. Indeed, intron 1 but not the promoter of cathepsin Q responded to p53-mediated transactivation (Fig. 3D). Moreover, mutation of the identified p53-binding site abolished its responsiveness to p53 (Fig. 3D). Taken together, our data uncovered an active genetic pro- gram, the p53-cathepsin Q axis, which initiates ‘‘programmed necrotic death’’ in response to DNA damage. ROS Cooperates with p53-Cathepsin Q to Execute DNA Damage- Induced Necrotic Death. Because cathepsin Q is homologous to cathepsin L and no specific cathepsin Q inhibitors are available, we used a cathepsin L inhibitor to chemically probe the cathepsin Q executed necrosis. Cathepsin L inhibitor, z-FY-CHO, significantly inhibited necrotic death in DKO cells despite the minor toxicity associated with the chemical (Fig. 3E). Of note, other cathepsin Fig. 1. DNA damage activates necrotic death in Bax and Bak DKO cells.
Details
-
File Typepdf
-
Upload Time-
-
Content LanguagesEnglish
-
Upload UserAnonymous/Not logged-in
-
File Pages6 Page
-
File Size-