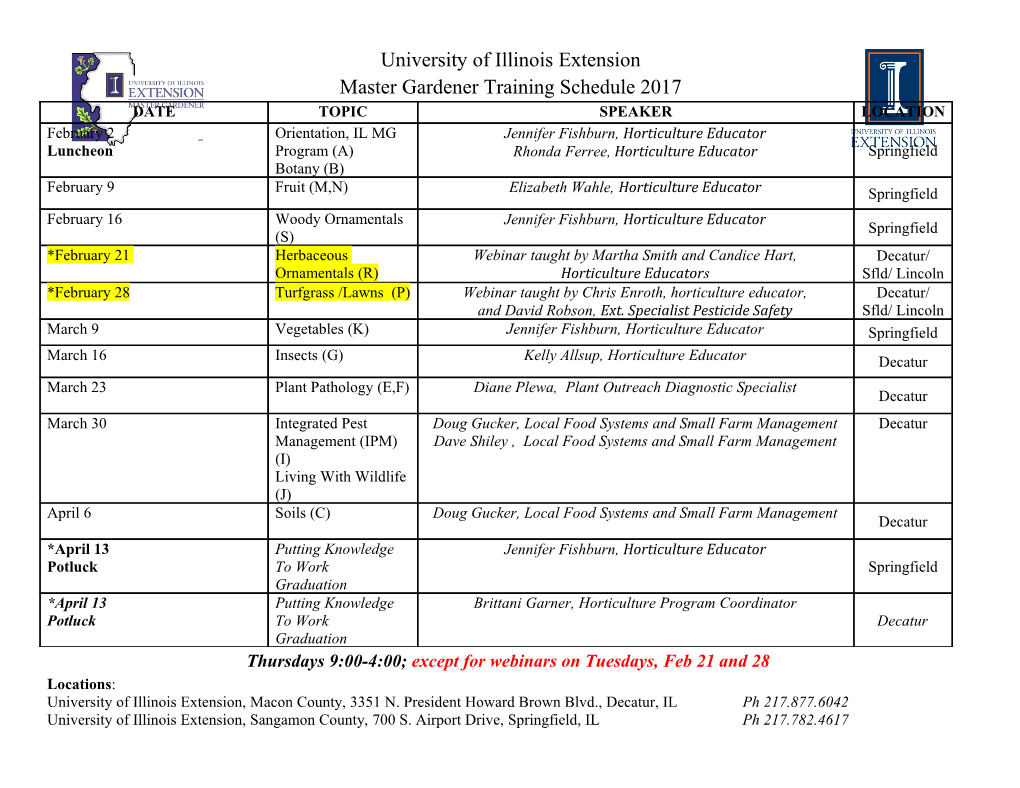
General Disclaimer One or more of the Following Statements may affect this Document This document has been reproduced from the best copy furnished by the organizational source. It is being released in the interest of making available as much information as possible. This document may contain data, which exceeds the sheet parameters. It was furnished in this condition by the organizational source and is the best copy available. This document may contain tone-on-tone or color graphs, charts and/or pictures, which have been reproduced in black and white. This document is paginated as submitted by the original source. Portions of this document are not fully legible due to the historical nature of some of the material. However, it is the best reproduction available from the original submission. Produced by the NASA Center for Aerospace Information (CASI) ( y 19E ,._ MASS SPECTROMETRY IN STRUCTURAL AND STEREOCHEMI£AL PROBLEMS CLXXVIII. The Electron-Impact Promoted Fragmentation of 1,2-Cyclohexene Oxide M. Karen Strong, Peter Brown, and Carl Djerassi (Department of Chemistry, Stanford University, Stanford, California, 94305 U.S.A.) Abstract. The mass spectrum of 1,2-Cyclohexene oxide and important data from the spectra of three deuterium labeled analogs have been recorded and mechanistic rationalizations are given for the most important frag- mentation processes observed. Multiple mechanisms are required to ex- plain the indicated peak shifts in the labeled analogs for many of the ions discussed, thus demonstrating again the care that needs to be exer- cised in the interpretation of the mass peaks of relatively simple mole- cules. Until recent years the mass spectral fragmentation patterns of epoxides have not been extensively studied. Detailed analyses of a series of aliphatic epoxides,l a,8-epoxyketones, 2 and aromatic alkene oxides have been reported. Discussions of the mass spectra of 8-phenylglycidic esters and amides and long chain aliphatic epoxy esters have also appeared. In addition, isolated spectra, partial analyses, and high resolution data for various other epoxides have been published. 6 No de- tailed analysis of the mass spectra of alicyclic epoxides has appeared to date, al- though a postulated fragmentation scheme for 1,2-epoxy-l-methylcyclohexane has been proposed, 1 and the spectra of this compound, 3,4-epoxymenthane, and 1,2-epoxymenthane 7b have been presented ar;d discussed with respect to the formation of m/e 69. There- fore, we decided to examine in detail the mass spectral fragmentation of the parent Cyclohexene oxide (I, Fig. 1 and 2) by means of deuterium labeled analogs (II-IV), medium resolution data, 8 and metastable findings for both the labeled and unlabeled For part CLXXVII see G. Eadon, J. Diekman, and C. Djerassi, submitted for publication. t Taken from the M.S. thesis of M. Karen Strong. f Present address: Department of Chemistry, Arizona State University, Tempe, Arizona 85281. N64;2 S g42 e c[ 1 NUM• wi - j nNRyl (J.AO[[/ (COD[! `i3333 n _ '^ Q^ /12 lNA[A CR - OR TMX WR AD NYM[[RI (CATEGORYL- _ _ 2 - cyclohexene oxides. D D D D D0 D o C>O D [:> aL D D D I II III IV The estimated deuterium content of each analog is listed in Table 1. Upon con- sideration of Figure 1, it became apparent that the deuterium incorporation in the three labeled analogs could not be calculated from the molecular ion region of the mass spectra of the epoxides. The very large M-1 peak (m/e 97), which apparently arises from random loss of a proton from all six carbons of the ring, necessitated a circumvention. A bracketing of isotopic purity was possible for epoxides III and IV, since the isotopic content of the precursor olefin and the acid hydrolysis product diol had been determined. The deuterium content of epoxide II, however, could be ascertained only from the precursor diol because the labeled cyclohexene was not iso- lated in pure form prior to epoxidation. Table 1. Isotopic content of the labeled 1,2-cyclohexene oxide. Compound %d0 %dl %d2 %d3 %d4 %d5 II - - 4t 8t 88t - III - - - - 97t-100 0*-3t IV 0*-1t 8*-llt 88t-92 - - - t Data calculated from the spectra of the corresponding diols. Data calculated from the spectra of the precursor olefins. Three tautomeric shifts, shown below, can be postulated to occur prior to frag- It is recognized that ions produced by the rearrangements suggested here may differ in energy from the corresponding ions produced by direct ionization of a molecule (for example, cyclohexanone). It is also recognized that these two energetically different ions may produce fragmentation patterns differing slightly in peak in- tensities. Nevertheless, the arguments presented here seem sufficiently valid to warrant consideration. - 3 - mentation. The first of these, labeled AA, has been postulated to occur in cleavages observed in aliphatic epoxides 1 and aromatic alkene epoxides. 3 In the present situation, this shift would give rise to the molecular ion of cyclohexanone, which has been studied in great.detail. 9 It has principal fragmentation peaks at m/e 42, We 55 (C3H3O), We 69, and m/e 70. The latter two peaks are present in 30% and 20% relative: abundance with respect to the base peak of m/e 55. In the spectrum of I, 41% of the m/e 55 peak is C3H3O and this portion could only partly arise from the indicated tautomeric shift and subsequent fragmentation, since the spectra of the deuterated analogs II-IV demonstrate shifts in addition to those explained by this process. Therefore this re- arrangement cannot explain the entire C3H3O portion of m/e 55, if indeed any frag- mentation occurs by this method. The intensity of the We 69 and m/e 70 peaks in the spectrum of cyclohexene oxide cannot be explained by this tautomeric shift AA, since, the relative contribution to either peak through such an intermediate would be minimal. Therefore, it would seem that cyclohexene oxide, a simple cyclic epoxide, does not fragment to any great degree via this route. The tautomeric shift BB results in a species corresponding to the molecular ion 10 of 2-cyclohexene-1-ol, whose mass spectrum is reproduced in Figure 3. The corapo- sition of the base peak of mass 70 is 100% C4H6O and is postulated to arise from the molecular ion by a retro-Diets-Alder fragmentation 11 (see scheme D). Medium reso- lution data (see Table 3) for the m/e 70 peak of cyclohexene oxide (27% relative abundance) show it to be 93% C 4H6O. Therefore, it is conceivable that part of the peak in the epoxide could a-:se through the ionized cyclohexenol intermediate. The entire peak cannot be attributed to this process, however, since the deuterated analogs display mass shifts in addition to those explained by the fragmentation of the tauto- meric species BB. Assuming that the fragmentation pattern of 2-cyclohexene-1-ol remains constant, the only other fragmentation peaks of I to which the tautomeric alcohol might contribute substantially would be We 55 and We q3. The ion of mass 55 in the spectrum 4 - of cyclohexenol is principally oxygen-containing, while that in the spectrum of cyclo- hexene oxide is 41% C H 0 (see Table 3). A metastable peak at m/e 43.2 in the un- 3 3 labeled spectrum (see Table 2) and appropriate metastable peaks in the spectra of the deuterium analogs II-IV substantiate a possible transition from the m/e 70 ion arising from the tautomeric cyclohexenol to the oxygen-containing ion of mass 55. The m/e 83 peak of cyclohexene oxide could result in part from the loss of methyl from the molecular ion of 2-cyclohexene-1-ol (see process B'), since the predicted frag- mentation is supported by the mass shifts observed in the labeled analogs. However, H 0t 0t BB —^ t bH CC CO this type of fragmentation is not the principal mode of formation of m/e 83, since the contribution of the tautomeric species to this peak would have as a maximum approx- imately one-third of the relative abundance of We 70 (27 units), clearly a minor (10%) contribution to the base peak of cyclohexene oxide. The third possible tautomeric shift CC would give rise to the molecular ion of tetrahydro-oxepin. The mass spectrum of this compound is unknown, and therefore, this rearrangement cannot be discounted or confirmed. The base peak of both the 70 eV spectrum (Fig. 1) and the low voltage spectrum (nominal voltage 12 eV, Fig. 2) is m/e 83. With the exception of the region around m/e 42, the spectra are quite similar. The only substantial difference is the intensity of the remaining peaks, which, as expected, decrease at low voltage. This decrease is fairly uniform, except for m/e 68 and m/e 56, which seem to increase with respect to their neighboring ions, indicating that these ions are probably formed by a primary - 5 - fragmentation process, or by a rearrangement in the molecular ion prior to cleavage. 12 Metastable peaks are observed in the high voltage spectrum of I for the transitions given in Table 2. The spectra of II-IV demonstrate metastable peaks corresponding to the loss of the species and/or deuterium labeled analogs of the species listed in Table 2. The composition of the important fragment ions, obtained on a dual-focusing MS-9 mass spectrometer, are given in Table 3. Table 2. Metastable Reaks and possible transitions for 1,2-cyclohexene oxide. Metastable peak observe' Transition Species lost 96.0 98 --> 97 H' 70.3 98 83 CH 3* 48.6 98 69 C2H5 47.2 98 68 CH2O 43.2 70 55 CH 3' 36.5 83--> 55 CO Table 3.
Details
-
File Typepdf
-
Upload Time-
-
Content LanguagesEnglish
-
Upload UserAnonymous/Not logged-in
-
File Pages23 Page
-
File Size-