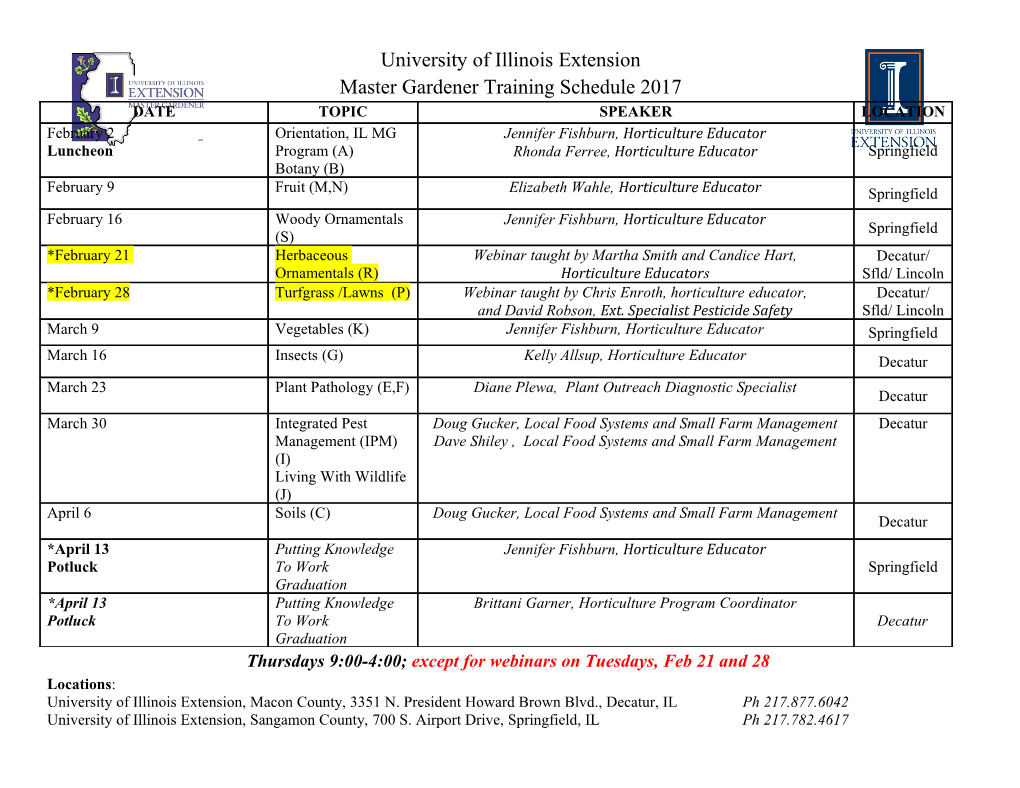
The ATLAS Experiment at the CERN Large Hadron Collider A 30 minute tour Peter Krieger University of Toronto P.Krieger, University of Toronto WNPPC'08, Banff, February 2008 0 The Standard Model of Particle Physics SU(3)C SU(2)L U(1)Y e μ Consistent with all existing experimental data BUT e L μ L L No Higgs yet u c t 19 free parameters (masses, couplings etc) three (?) generations of fundamental fermions d s b L L L Hierarchy problem (need Supersymmetry) Charge ratios of quarks and leptons (GUTs) No gravity (need string theory ?) ± 0 0 W ,Z Higgs H A Number candidates for physics beyond the SM g Expected mass scale for new physics ~ 1 TeV P.Krieger, University of Toronto WNPPC'08, Banff, February 2008 1 Beyond the Standard Model Hierarchy problem: there are two fundamental energy scales that we know -17 of: the electroweak scale and the Planck scale: MEW / Mplanck 10 Naturalness problem: radiative corrections to the mass of a fundamental scalar (e.g. the Higgs) scale like 2 where is the energy scale to which the theory remains valid. This yields a fine-tuning problem for the Higgs mass unless: Could be gravity if there a) There is new physics at the ~ TeV energy scale are extra dimensions b) There is some symmetry protecting the Higgs mass against large radiative corrections (Supersymmetry) If the Higgs is not discovered with a mass < 800 GeV, expect the dynamics of WW, ZZ scattering to reveal new physics at this energy scale We MUST see something at LHC energies P.Krieger, University of Toronto WNPPC'08, Banff, February 2008 2 Vector Boson Scattering 2 Cross-section grows with s E CM . Eventually violates unitarity (probability) unless there are additional processes. Need to add with M H 1 TeV P.Krieger, University of Toronto WNPPC'08, Banff, February 2008 3 Constraints on the Higgs Mass LEP Direct Search Limit MH > 114 GeV Low mass Higgs is favoured P.Krieger, University of Toronto WNPPC'08, Banff, February 2008 4 Supersymmetry Each SM boson (fermion) has a fermionic (bosonic) supersymmetric partner with IDENTICAL MASS and Standard Model COUPLINGS leptons sleptons ~ ~ ~ e μ e μ gauginos higgsinos gluinos ~ ~ ~ ± ~ ± 0 ~ 0 ~ e μ e μ W W h h g g 0 ~ 0 0 ~ 0 ~ Z Z H H u c t u~ ~c t ~ 0 ~ 0 ~ ~ A A d s b d ~s b ~ H ± H ± quarks squarks Obviously we do not see such particles. So we say SUSY is a broken symmetry. However, most motivations for SUSY require a mass scale of less than about 1 TeV P.Krieger, University of Toronto WNPPC'08, Banff, February 2008 5 Force Unification with and without SUSY Weak-scale SUSY seems to allow for force unifications at high energy (running of coupling constants with energy): No SUSY with SUSY Assumes a TeV scale SUSY particle mass spectrum P.Krieger, University of Toronto WNPPC'08, Banff, February 2008 6 R-parity R-Parity is a quantum number which distinguishes SM and supersymmetric particles R = (1)3(BL)+2S Most supersymmetric models assume R-Parity Conservation This has two important consequences: Supersymmetric particles must be produced in pairs There must be some Lightest Supersymmetric Particle or LSP ~0 This LSP is usually the lightest neutralino 1 which can be a good Cold Dark Matter candidate. This leads to an experimental signature of large transverse missing energy. In the case of pair production of squarks and gluinos at the LHC, the standard signature is jets + missing energy P.Krieger, University of Toronto WNPPC'08, Banff, February 2008 7 Cosmological Issues Evidence is that dark matter is predominantly “cold”, e.g. non-relativistic. Popular candidate is the WIMP (Weakly Interacting Massive Particle). The LSP can be a very good candidate for this. P.Krieger, University of Toronto WNPPC'08, Banff, February 2008 8 Some Basic Collider Physics How does one calculate the rate for some physics process at a collider ? M = sum of all contributing processes, here for e+e- W+W- Define cross-section |M|2 units of (length)2 f bunch crossing frequency Define luminosity L N A ~Number of ~ cross-sectional size of the beams particle bunches times numbers of particles in each Instantaneous production rate N = L bunch P.Krieger, University of Toronto WNPPC'08, Banff, February 2008 9 Hadron Colliders vs Electron Positron Colliders Bending a charged particle in a magnetic field costs energy emitted in the form of synchrotron radiation: 2 2 4 4 e 1 4 E = • or E 3 m4 For fixed radius machine (i.e. in the LEP tunnel at CERN with = 6.28km) synchrotron radiation loss for protons is less that that for electrons by the amount Cannot (feasibly) build electron synchrotrons 4 m of arbitrarily high energy. Need either: e 1013 hadron collider mp linear electron positron collider P.Krieger, University of Toronto WNPPC'08, Banff, February 2008 10 The Large Hadron Collider at CERN Proton-proton collider installed into the 27km circumference LEP ring at CERN in Geneva Switzerland: pp centre-of-mass energy of 14 TeV constituent centre-of-mass energies ~ 1-2 TeV luminosity of 1033 cm-2s-1 (low luminosity) 1034 cm-2s-1 (high luminosity) proton bunch spacing of 25 ns (40MHz collision frequency) Physics goals: whatever TeV-scale physics is there to be discovered • Higgs boson • Extended gauge theories • Supersymmetry • Compositeness • Extra dimensions • Low-scale gravity P.Krieger, University of Toronto WNPPC'08, Banff, February 2008 11 CERN Aerial View P.Krieger, University of Toronto WNPPC'08, Banff, February 2008 12 LHC Accelerator Chain P.Krieger, University of Toronto WNPPC'08, Banff, February 2008 13 The CERN Large Hadron Collider P.Krieger, University of Toronto WNPPC'08, Banff, February 2008 14 Cutaway View LHC/ATLAS (Graphic) P.Krieger, University of Toronto WNPPC'08, Banff, February 2008 15 The LHC Tunnel P.Krieger, University of Toronto WNPPC'08, Banff, February 2008 16 Quadrupole Magnets from Canada P.Krieger, University of Toronto WNPPC'08, Banff, February 2008 17 The proton-proton total cross-section = 1034 cm-2/s · 100mb · 10-27cm-2/mb = 109/s L tot LHC 109/s · 25ns 25 interactions / bunch crossing This is referred to as “pileup” P.Krieger, University of Toronto WNPPC'08, Banff, February 2008 18 Min-Bias Events at High Luminosity (1034 cm-2s-1) High event rate results in large detector occupancies High charged particle multiplicity visible in tracking detector ATLAS H P.Krieger, University of Toronto WNPPC'08, Banff, February 2008 19 Production cross-sections at the LHC P.Krieger, University of Toronto WNPPC'08, Banff, February 2008 20 Higgs Branching Fractions vs MH In terms of discovery potential, MH matters a lot. Low mass is tricky due to huge QCD backgrounds. P.Krieger, University of Toronto WNPPC'08, Banff, February 2008 21 Higgs Discovery Significance at ATLAS 1 L dt = 30 fb Vector boson fusion important in the low ATLAS mass region for example, VBF H + P.Krieger, University of Toronto WNPPC'08, Banff, February 2008 22 LHC luminosity profile and physics reach early physics O(1fb-1) P.Krieger, University of Toronto WNPPC'08, Banff, February 2008 23 Collider Detectors Events reconstructed based on particles stable enough to be detected Tracking EM Hadronic Muon Detector Detector Calorimeters 0 nK, L e± μ ± ± , p 0 nK, L Charged e±, muons particle momentum, -------Hadrons, jets---------- particle ID P.Krieger, University of Toronto WNPPC'08, Banff, February 2008 24 Calorimeters vs Magnetic Spectrometers A calorimeter measures particle / jet energies via total energy deposition in the device e.g. absorption of entire particle / jet energy through a showering process (EM or hadronic). Magnetic spectrometers measure particle momenta via curvature in a known magnetic field (usually solenoidal, but also toroidal in the case of the ATLAS muon spectrometer). For a given design, the depth of a calorimeter capable of providing full containment of high energy particles scales like ln(E). For a magnetic spectrometer, the resolution p/p, for a given detector size, scales like sqrt(E). Magnetic spectrometers must get larger at higher energies, to achieve the same momentum resolution. In ATLAS, most of the detector volume is occupied by the muon spectrometer. P.Krieger, University of Toronto WNPPC'08, Banff, February 2008 25 The ATLAS Detector people shown for scale P.Krieger, University of Toronto WNPPC'08, Banff, February 2008 26 ATLAS Event Slice P.Krieger, University of Toronto WNPPC'08, Banff, February 2008 27 The ATLAS Canada Collaboration Alberta 42 University/Lab Physicists Carleton Montreal 150 People, including engineers, McGill Simon technicians and students Fraser Regina 20 Undergraduate students Toronto 60 Graduate Students TRIUMF 20 Postdocs UBC Victoria York Other Important Activities High Level Trigger Focus has been on LAr Calorimetry ATLAS Computing TRT Electronics Four NSERC funded projects: ATLAS Upgrades (SLHC) Hadronic Endcap Calorimeter Beam Conditions Monitors Beam Testing / Analysis Hadronic Forward Calorimeter Calorimeter Calibration Endcap Signal Cryogenic Feedthroughs Physics Studies / Analysis Radiation Hardness Studies Front-End Board Electronics Pixel Testing and Assembly P.Krieger, University of Toronto WNPPC'08, Banff, February 2008 28 ATLAS Collaboration (Status October 2007) 37 Countries 167 Institutions 2000 Scientific Authors total (1600 with a PhD) CANADA: ~ 4% of collaboration Albany, Alberta, NIKHEF Amsterdam, Ankara, LAPP Annecy, Argonne NL, Arizona, UT Arlington, Athens, NTU Athens, Baku, IFAE Barcelona, Belgrade, Bergen,
Details
-
File Typepdf
-
Upload Time-
-
Content LanguagesEnglish
-
Upload UserAnonymous/Not logged-in
-
File Pages57 Page
-
File Size-