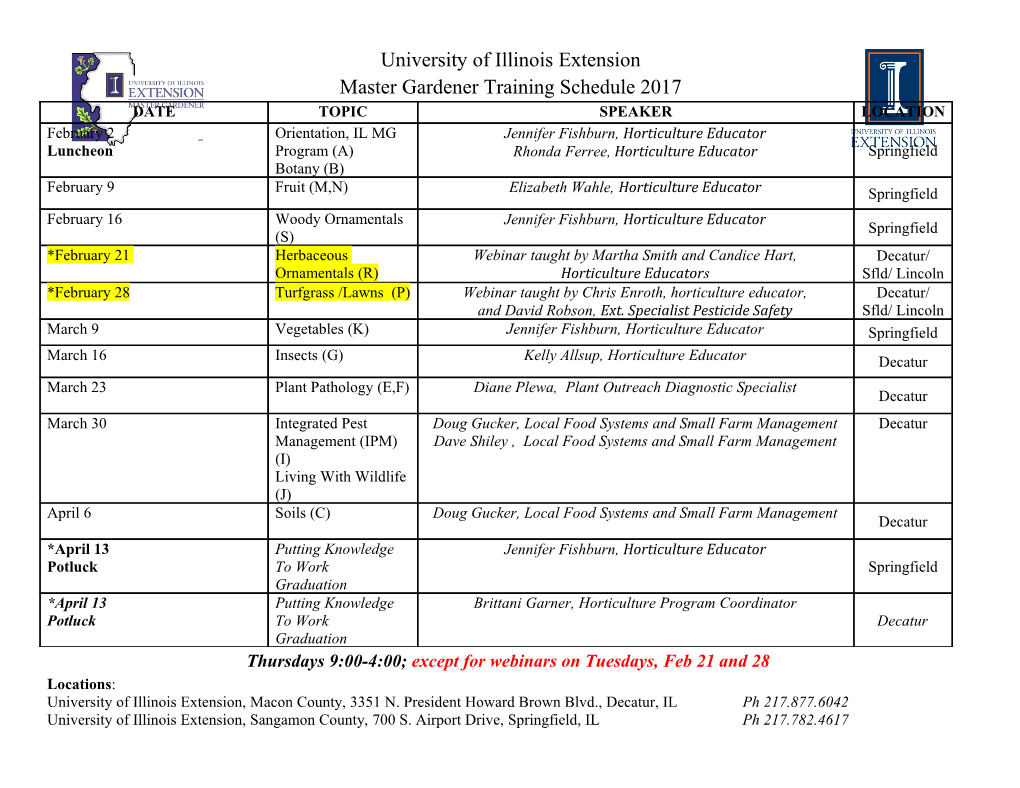
Biological Charge Transfer in Redox Regulation and Signaling by Ruijie Darius Teo Department of Chemistry Duke University Date:_______________________ Approved: ___________________________ David Beratan, Advisor ___________________________ Patrick Charbonneau ___________________________ Agostino Migliore ___________________________ Kenichi Yokoyama Dissertation submitted in partial fulfillment of the requirements for the degree of Doctor of Philosophy in the Department of Chemistry in the Graduate School of Duke University 2020 i v ABSTRACT Biological Charge Transfer in Redox Regulation and Signaling by Ruijie Darius Teo Department of Chemistry Duke University Date:_______________________ Approved: ___________________________ David Beratan, Advisor ___________________________ Patrick Charbonneau ___________________________ Agostino Migliore ___________________________ Kenichi Yokoyama An abstract of a dissertation submitted in partial fulfillment of the requirements for the degree of Doctor of Philosophy in the Department of Chemistry in the Graduate School of Duke University 2020 i v Copyright by Ruijie Darius Teo 2020 Abstract Biological signaling via DNA-mediated charge transfer between high-potential [4Fe4S]2+/3+ clusters is widely discussed in the literature. Recently, it was proposed that for DNA replication on the lagging strand, primer handover from primase to polymerase α is facilitated by DNA-mediated charge transfer between the [4Fe4S] clusters housed in the respective C-terminal domains of the proteins. Using a theoretical-computational approach, I established that redox signaling between the clusters in primase and polymerase α cannot be accomplished solely by DNA-mediated charge transport, due to the unidirectionality of charge transfer between the [4Fe4S] cluster and the nucleic acid. I extended the study by developing an open-source electron hopping pathway search code to characterize hole hopping pathways in proteins and nucleic acids. I used this module to analyze protective hole escape routes in cytochrome p450, cytochrome c oxidase, and benzylsuccinate synthase. Next, I used the module to analyze molecular dynamics snapshots of a mutant primase, where the Y345C mutation (found in gastric tumors) attenuates charge transfer between the [4Fe4S] cluster and nucleic acid, which in turn, could disrupt the signaling process between primase and polymerase α. In another protein-nucleic acid system, I found that charge transfer in the p53-DNA complex plays an important role for p53 to differentiate Gadd45 DNA and p21 DNA in metabolic pathway regulation. Using density functional theory calculations iv on molecular dynamics snapshots, I found that hole transfer (HT) from Gadd45 DNA to the proximal cysteine residue in the DNA-binding domain of p53 is preferred over HT from p21 DNA to cysteine. This preference ensures that the p21 DNA remains bound to the transcription factor p53 which induces the transcription of the gene under cellular oxidative stress. This dissertation concludes with a study that demonstrates similar electron conductivities between an artificial nucleic acid, 2'-deoxy-2'-fluoro- arabinonucleic acid (2’F-ANA), and DNA. Compared to DNA, 2’F-ANA offers the additional benefit of chemical stability with respect to hydrolysis and nuclease degradation, thereby promoting its use as a sensor in biological systems and cellular environments. v Contents Abstract .......................................................................................................................................... iv List of Tables .................................................................................................................................. x List of Figures ............................................................................................................................. xiii Acknowledgements ................................................................................................................... xvi 1. Introduction ............................................................................................................................... 1 1.1 Charge Transfer in Proteins ............................................................................................ 2 1.2 Charge Transfer in DNA ................................................................................................. 4 1.3 Charge Transfer in Protein-DNA Systems .................................................................... 5 1.4 Aims and Objectives ........................................................................................................ 7 2. Classical Marcus Theory .......................................................................................................... 8 2.1 Charge Transfer Mechanisms ....................................................................................... 11 2.1.1 Tunneling .................................................................................................................... 12 2.1.2 Superexchange ........................................................................................................... 13 2.1.3 Flickering Resonance ................................................................................................ 14 2.1.4 Hopping ...................................................................................................................... 16 3. Electronic Couplings ............................................................................................................... 17 3.1 Empirical/Semi-Empirical Models ............................................................................... 17 3.1.1 Hopfield Model .......................................................................................................... 17 3.1.2 Pathway Tunneling Model ....................................................................................... 18 3.1.3 Average Packing Density Model ............................................................................. 19 vi 3.2 Electronic Structure Models .......................................................................................... 20 3.2.1 Generalized Mulliken-Hush Method ..................................................................... 20 3.2.2 Effective Hamiltonians ............................................................................................. 21 3.2.2.1 Localized Orbitals .............................................................................................. 21 3.2.3 Constrained Density Functional Theory (CDFT) .................................................. 22 3.2.4 Orbital Splitting ......................................................................................................... 25 4. Reorganization Energy ........................................................................................................... 26 4.1 Marcus’ Two-Sphere Model .......................................................................................... 26 4.1.1 Inner-Sphere Reorganization Energy ..................................................................... 27 4.1.2 Outer-Sphere Reorganization Energy .................................................................... 29 5. Molecular Dynamics ............................................................................................................... 30 5.1 MD Force Fields .............................................................................................................. 31 5.1.1 Seminario Method for Calculating Force Constants ............................................ 33 5.1.2 Atomic Charges for Coulomb Interactions ............................................................ 34 5.2 Integration Algorithms for System Evolution ............................................................ 35 5.3 Solvent Representation .................................................................................................. 37 5.4 Periodic Boundary Conditions ..................................................................................... 39 5.5 Temperature/Pressure Coupling Algorithms ............................................................. 40 6. Kinetic Models and Master Equations for Charge Transfer Dynamics .......................... 43 7. Charge Transfer in the Human Primosome ........................................................................ 46 7.1 Methodology ................................................................................................................... 49 7.1.1 Docking and Modeling ............................................................................................. 49 vii 7.1.2 Redox Potentials ........................................................................................................ 51 7.1.3 Reorganization Energies ........................................................................................... 52 7.1.4 Electronic Couplings ................................................................................................. 53 7.1.5 Kinetic Model ............................................................................................................. 56 7.2 Unidirectionality ............................................................................................................. 57 7.3 Concluding Remarks ...................................................................................................... 63 8. Mapping Hole Hopping Pathways in Proteins .................................................................
Details
-
File Typepdf
-
Upload Time-
-
Content LanguagesEnglish
-
Upload UserAnonymous/Not logged-in
-
File Pages194 Page
-
File Size-