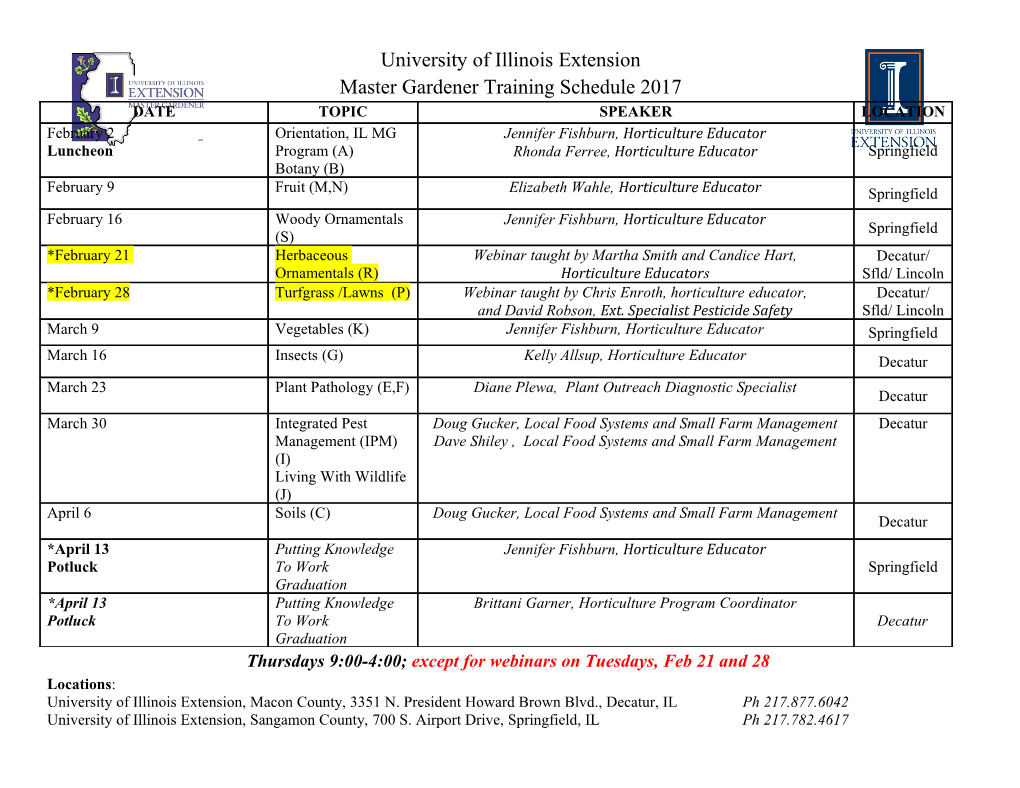
In: Conners, Terrance E.; Banerjee, Sukit, eds. Surface analysis of paper. Boca Raton, FL: CRC Press, Inc.: 152-181; 1995. Chapter 8 8 Raman Spectroscopy Umesh P. Agarwal and Rajai H. Atalla USDA Forest Products Laboratory, Madison, Wisconsin, U.S.A. INTRODUCTION Since the discovery of the Raman effect in 1928, Raman spectroscopy has been a field of major advances. In the early years, the technique was primarily used to study vibrational states of simple molecules. This was due to the unavailability of intense monochromatic light sources and the lack of suitable optical components and detectors. However, with the discovery of lasers in 1960s and the development of high-throughput mono- chromators and sensitive detectors, Raman spectroscopy has been increasingly applied to solve problems of both fundamental and technological interest. In the area of wood, pulp, and paper research, however, Raman spectroscopy has not been used very much. Primarily, this seems to be due to the fact that in conventional Raman spectroscopy sample-generated laser-induced fluorescence (LIF) overwhelms the Raman signal. It was only when means to suppress the LIF contribution (to a Raman spectrum) were found, that useful spectra were obtained. Additional factors responsible for limited Raman use in pulp and paper research were instrumentation costs, spectrum acquisition time, the lack of trained personnel, and a perception that Raman and infrared (IR) spectroscopies provide the same information. In reality, the two techniques are complementary. With the development of near-IR FT Raman spectroscopy, LIF is no longer a problem for most paper and lignocellulosic samples. This is evident from the fact that even unbleached kraft pulps (strongly fluorescent in conventional Raman) have been success- fully analyzed using the near-IR FT Raman approach.1,2 Another advantage with the FT technique is that the time needed to acquire a spectrum has been drastically reduced. With these developments behind us, no barriers exist to using Raman spectroscopy and its application in pulp and paper research is expected to increase. Indeed, FT Raman systems are now available in industrial laboratories affiliated with the pulp and paper industry. None of the experimental difficulties encountered in complex lignocellulosic systems are present when cellulose and lignin models are studied using conventional Raman 152 1995 by CRC Press, Inc. Raman Spectroscopy 153 spectroscopy. 3-10 Normal coordinate analyses of such models have been carried out to provide a conceptual framework for interpretation of spectra of cellulose and lignin. Although such studies contributed significantly to the Raman studies of wood, pulp, and paper, additional studies of models and other simpler systems will be needed in the future to solve problems using Raman spectroscopy. PRINCIPLES The phenomena underlying Raman spectroscopy can be described with reference to infrared spectroscopy as shown schematically in Figure 1. The primary event in infrared absorption is the transition of a molecule from a ground state (M) to a vibrationally excited state (M*) by absorption of an infrared photon with energy equal to the differ- ence between the energies of the ground and the excited states. The reverse process, infrared emission, occurs when a molecule in the excited state (M*) emits a photon during the transition to a ground state (M). In infrared spectroscopy, one derives information by measuring the frequencies of infrared photons a molecule absorbs and interpreting these frequencies in terms of the characteristic vibrational motions of the molecule. In complex molecules, some of the frequencies are associated with functional groups that have characteristic localized modes of vibration. 154 Surface Analysis of Paper As shown in Figure 1, the same transitions between molecular vibrational states (M) and (M*) can result in Raman scattering. A key difference between the Raman and infrared processes is that the photons involved are not absorbed or emitted but rather shifted in frequency by an amount corresponding to the energy of the particular vibra- tional transition. In the Stokes process, which is the parallel of absorption, the scattered photons are shifted to lower frequencies as the molecules abstract energy from the exciting photons; in the anti-Stokes process, which is parallel to emission, the scattered photons are shifted to higher frequencies as they pick up the energy released by the molecules in the course of transitions to the ground state. In addition, a substantial number of the scattered photons are not shifted in frequency. The process which gives rise to these photons is known as Rayleigh scattering. This scattering arises from density variations and optical heterogeneities and is many orders of magnitude more intense than Raman scattering. Figure 1 depicts another major difference between Raman scattering and infrared processes. To be active in the infrared spectra, transitions must have a change in the molecular dipole associated with them. For Raman activity, in contrast, the change has to be in the polarizability of the molecule. These two molecular characteristics are qualitatively inversely related. A Raman spectrum is obtained by exposure of a sample to a monochromatic source of exciting photons, and measurement of the light intensity at the frequencies of the scattered light. Because the intensity of the Raman scattered component is much lower than that of the Rayleigh scattered component, filters and diffraction gratings are used to suppress the latter component. A highly sensitive detector is required to detect weakly scattered Raman photons. In conventional Raman spectroscopy where visible laser radiation is used, the exciting photons are typically of much higher energies than those of the fundamental vibrations of most chemical bonds or systems of bonds, usually by a factor ranging from about 6 for O-H and C-H bonds to about 200 for bonds between very heavy atoms, as for example in I2. The 514.5 and 488 nm lines from an argon ion laser are often used as exciting frequencies. In contrast, in the case of FT Raman spectroscopy, excitation with a laser is usually carried out in the near-IR region (1064 nm). The energies of the exciting photons are higher by factors that are one-half or less of the above-mentioned values. Another point to consider in near-IR excited Raman is that, for some samples, absorption of Raman scattered photons due to overtone and combination modes of molecular vibra- tions can occur. An important difference between the conventional and near-IR FT approaches is the difference in the scattering efficiencies at the absolute Raman frequencies. Because Raman scattering is directly proportional to the fourth power of the absolute frequency of a Raman line, in the FT approach, Raman scattering is lower by factors anywhere between 18 to 53 when compared to the 514.5 nm excited spectra. However, this is not Raman Spectroscopy 155 a problem because of the “multiplex” and throughput advantages of the interferometer in the FT approach. 11 lNSTRUMENTATlON CONVENTIONAL RAMAN A number of stages are involved in the acquisition of a Raman spectrum using a conven- tional approach. A sample is mounted in the sample chamber and laser light is focused on it with the help of a lens. Generally, liquids and solids are sampled in a Pyrex capillary tube. Solids are sampled either as pellets or are examined directly without any sample preparation. The scattered light is collected using another lens and is focused at the entrance slit of the monochromator. Monochromator slit widths are set for the desired spectral resolution. The monochromator effectively rejects Raleigh scattering and stray light and serves as a dispersing element for the incoming radiation; sometimes more than one monochromator is used to obtain high resolution and/or better suppression of the Rayleigh line. The light leaving the exit slit of the monochromator is collected and focused on the surface of a detector. This optical signal is converted to an electrical signal withn the detector and further manipulated using detector electronics. In a conventional Raman system using a photomultiplier tube (PMT) detector, the light intensity at various frequencies is measured by scanning the monochromator. In contrast, when a multichannel detector is used a spectral range is simultaneously recorded. A typical conventional Raman system consists of the following basic components: i) an exatation source, usually a visible-light laser; ii) optics for sample illumination and collection of sample-scattered light; iii) a monochromator; and iv) a signal processing system consisting of a detector and a data processing unit. A diagram showing the various components of a Raman spectrometer is shown in Figure 2. 156 Surface Analysis of Paper Although argon and krypton ion lasers are routinely used for sample excitation, for special purposes UV lasers have also been used.12 The choice of a suitable mono- chromator is dictated both by the spectral resolution desired and by the spectral range in which the spectrometer is to be used. A number of detector types exist in conventional Raman spectroscopy. For the scanning instruments, the most frequently used detector is the PMT. More recently, however, multichannel detectors mounted in the plane of the exit slit have been increasingly used. These consist of charge coupled devices and silicon photodiode arrays. These are especially desirable if high resolution is not required and rapid spectral acquisition is needed. FT RAMAN The instrumentation needs of conventional and FT Raman techniques are different. Although the former technique has been available for quite some time and has benefited from developments, FT Raman instruments have only recently become available. In fact, the feasibility of FT Raman was demonstrated for the first time in 1986.13 It is likely, therefore, that further developments will improve the available FT instrumentation. Conventional Raman systems are based upon the use of dispersive monochromators of various kinds. FT Raman instruments, on the other hand, are built around an interfer- ometer.
Details
-
File Typepdf
-
Upload Time-
-
Content LanguagesEnglish
-
Upload UserAnonymous/Not logged-in
-
File Pages32 Page
-
File Size-