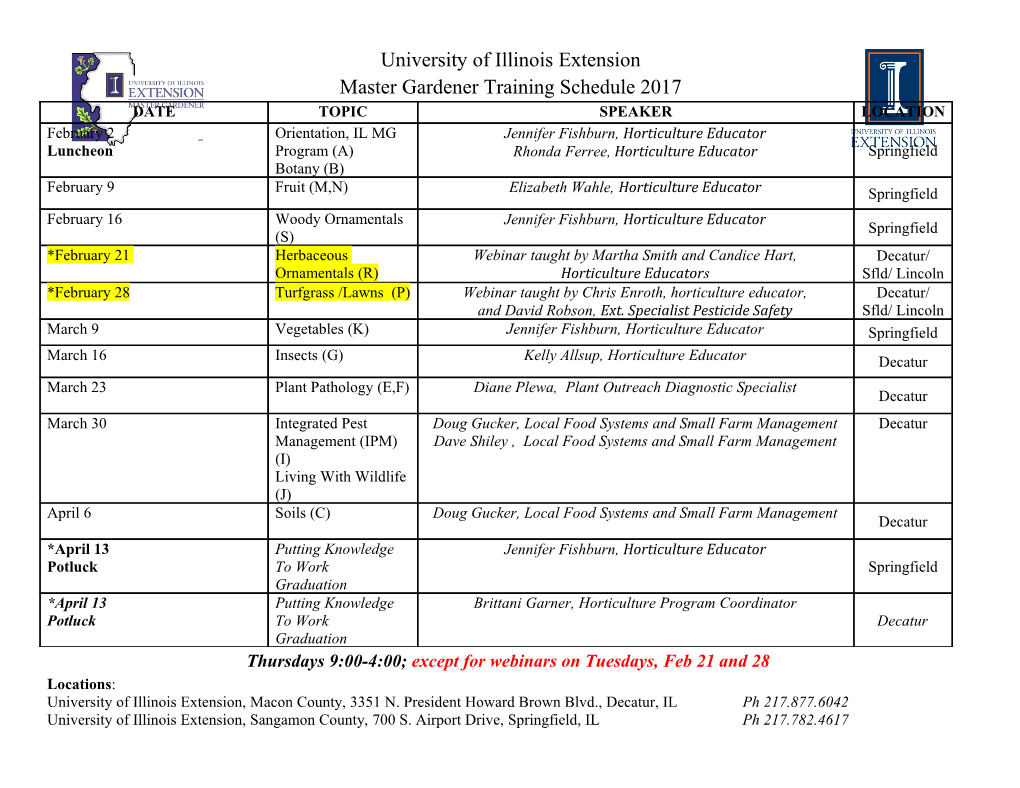
Catling, D.C. (2015) Planetary atmospheres, in G. Schubert (ed.) Treatise on Geophysics, 2nd Ed., vol. 10, Oxford, Elsevier, p.429-472. 10.13 Planetary Atmospheres DC Catling, University of Washington, Seattle, WA, USA ã 2015 Elsevier B.V. All rights reserved. 10.13.1 Introduction and Scope 429 10.13.2 Composition and Vertical Structure 430 10.13.2.1 The Observed Compositional Diversity of Atmospheres 430 10.13.2.2 Nomenclature for the Vertical Structure of Atmospheres 432 10.13.2.3 The Equation of State, Atmospheric Composition, and the Hydrostatic Equation 434 10.13.2.4 Convection and Stability 435 10.13.2.5 Condensables and the Clausius–Clapeyron Equation 437 10.13.3 Radiation 438 10.13.3.1 Planetary Energy Balance, Blackbody Radiation, and the Greenhouse Effect 438 10.13.3.2 Radiative Transfer: Shortwave and Infrared 441 10.13.3.3 Optical Thickness and Thinness 444 10.13.3.4 Radiative–Convective Equilibrium 445 10.13.3.5 Absorption and Emission by Atmospheric Gases 450 10.13.3.5.1 Rotational transitions 451 10.13.3.5.2 Vibrational transitions 451 10.13.3.5.3 Collision-induced absorption 451 10.13.3.5.4 Broadening 451 10.13.3.5.5 Continuum 452 10.13.3.5.6 Numerical radiative transfer 452 10.13.4 Photochemistry 452 10.13.4.1 Basic Principles of Chemical Kinetics 452 10.13.4.2 Why Free Radicals Are So Important 453 10.13.4.3 Photolysis 453 10.13.4.4 Earth’s Atmospheric Composition and Chemistry 454 10.13.4.5 CO2 Atmospheres: Venus and Mars 455 10.13.4.6 Reducing Atmospheres: Giant Planets and Titan 455 10.13.5 Atmospheric Dynamics 455 10.13.5.1 Equations of Motion 455 10.13.5.2 Balance Regimes: Geostrophic, Cyclostrophic, and Thermodynamic 457 10.13.5.2.1 Geostrophic balance 457 10.13.5.2.2 Cyclostrophic balance 457 10.13.5.2.3 Thermodynamic balance 458 10.13.5.3 Zonal Mean Meridional Circulation: Hadley Cells and Thermally Driven Jets 458 10.13.5.4 Eddy-Driven Jet Streams 461 10.13.5.5 Planetary Waves and Barotropic and Baroclinic Instabilities 462 10.13.5.6 Deep and Shallow Atmospheres on Giant Planets 464 10.13.5.7 Buoyancy Waves and Thermal Tides 465 10.13.5.8 Superrotation 465 10.13.6 Outstanding Questions 466 Acknowledgments 467 References 467 10.13.1 Introduction and Scope a new frontier that should reveal a larger diversity of atmospheres (Seager, 2010; Seager and Deming, 2010; Seager et al., 2010). But The enormous topic of planetary atmospheres ranges from the at the moment, only a little can be said about exoplanet atmo- origin of atmospheres to the fluid dynamics of small weather spheres with any certainty. Currently, observational data from systems, so here, I restrict the scope to broad essentials at a level such atmospheres are arguably much sparser than data were for suitable for a PhD research student. I assume knowledge of atmospheres in our solar system before the Space Age. Rather calculus, plus basic physics and chemistry, but little else. The than speculate, I concentrate on key principles associated with audience could also include researchers in allied fields – for the planetary atmospheres that we know best. In fact, these are example, planetary geologists, geochemists, or astronomers. what will be needed to interpret exoplanet atmospheres correctly I focus on atmospheres in the solar system. Exoplanets represent (see Chapter 10.21). Treatise on Geophysics, Second Edition http://dx.doi.org/10.1016/B978-0-444-53802-4.00185-8 429 Treatise on Geophysics, 2nd edition, (2015), vol. 10, pp. 429-472 Author's personal copy 430 Planetary Atmospheres Until the last 5 years or so, introductory syntheses about atmospheres (Moses et al., 2011) or early Mars (Zahnle et al., atmospheres were mostly confined to the Earth (e.g., 2008)), although we do not have an example in the modern Houghton, 2002; Wallace and Hobbs, 2006), with a few excep- solar system, and such an atmosphere would likely contain tions (Chamberlain and Hunten, 1987; Yung and DeMore, considerable H2 as well. The atmosphere of the early Earth was 1999), but now, the coverage of planetary atmospheres is also reducing, albeit weakly, before its oxygenation at 2.4 Ga more available. A primer by Ingersoll (2013) is a good read (Ga giga anna billions of years ago) (Catling, 2014; Lyons ¼ ¼ for all levels, while Taylor (2010) provides a useful introduc- et al., 2014). In contrast, oxidizing atmospheres are relatively tion aimed at mid-level undergraduates. At the research level, poor in reducing hydrogen-bearing gases and include modern general texts are Pierrehumbert (2010), Sanchez-Lavega Earth and Mars (Table 2). Molecular O2 has a volume abun- (2010), and Catling and Kasting (in press). In a more special- dance of 21 and 0.15% on Earth and Mars, respectively, which ized area, Yung and Demore (1999) provided a detailed mono- greatly exceeds the amount of hydrogen-bearing reducing gases graph on photochemistry. Recent papers have also reviewed of 1.8 ppmv (parts per million by volume) CH on Earth 4 the probable atmospheric circulation regimes of exoplanets (Hartmann et al., 2013) and 15 5 ppmv H on Mars Æ 2 (Showman et al., 2010, 2013). (Krasnopolsky and Feldman, 2001). Venus is unusual. The The structure of this review is as follows. In order to discuss upper atmosphere of Venus is oxidizing, with a tenuous atmospheres, I start with an overview of composition and ozone (O3) layer at 90–105 km altitude on the nightside basic physical properties, including the layers of atmospheres. (Montmessin et al., 2011), while the atmosphere below Thus, Section 10.13.2 describes structure and thermodynam- 20 km altitude is arguably weakly reducing because sulfur is ics. Atmospheric composition determines physical properties, converted into various reduced forms (Krasnopolsky and which in turn affects climate through the greenhouse effect. Pollack, 1994). Noting sulfur oxidation numbers in square Aplanet’sclimaterequiresagraspoftheradiativetransferof brackets, these sulfur species include sulfur dioxide (SO2) energy, which is discussed in Section 10.13.3.Abriefoverview [+4], carbonyl sulfide (OCS)[ 2], hydrogen sulfide (H2S) À of the photochemistry of atmospheres is then given in [ 2], and elemental sulfur allotropes of 1–8 atoms (S–S )[0]. À 8 Section 10.13.4 to provide an appreciation of the globally The OCS is sourced from weathering of sulfides on the surface, averaged bulk composition and vertical distribution of con- or volcanic outgassing, or the reduction of sulfur by carbon stituents. However, atmospheric motions transport heat and monoxide (e.g., S2 +CO), which is present at 20 ppmv below constituents, including condensable species, and are indis- 20 km (Fegley, 2014). Unlike Earth and Mars, O2 is absent in pensable for several aspects of climate and chemistry. Princi- Venus’ atmosphere with an upper limit of <0.3 ppmv. ples of atmospheric motion are described in Section 10.13.5. Broadly speaking, planetary formation theories from a Together, these various topics – thermodynamics, radiation, Sun-like nebula (reviewed by Chambers, 2014) favor more chemistry, and dynamics – provide the essentials of planetary hydrogen-rich atmospheres far from a star and more oxidizing atmospheres. Finally, in Section 10.13.6,Iverybrieflynote atmospheres nearby, but this can be confounded in an some key outstanding questions. extrasolar system if a planet’s orbital distance changes in plan- One topic is missing: atmospheric origin and evolution. etary migration. Also, the very early rocky planets in our own This subject is of profound interest but involves consider- solar system may have had hydrogen-rich atmospheres able geochemistry and familiarity with all the topics of (Abe, 2011). this review, so the reader is referred to Catling and Kasting Redox also indicates the possible chemistry of aerosols, (in press) that comprehensively reviews this area (see which are fine particles found in atmospheres. In general, Chapter 10.14). oxidizing atmospheres with sulfurous gases tend to produce sulfate aerosols at altitude (McGouldrick et al., 2011), such as in the Earth’s stratosphere (Crutzen, 1976; Robock, 2000), on 10.13.2 Composition and Vertical Structure Venus (Thompson et al., 2011), or on Mars when it was volcanically active (Settle, 1979; Smith et al., 2014; Tian 10.13.2.1 The Observed Compositional Diversity et al., 2010). In contrast, reducing atmospheres generate of Atmospheres hydrocarbon aerosols, including the giant planets (Irwin, A physically meaningful classification of the composition of 2009; Moses et al., 2004; West et al., 1991, 2004, 2009), planetary atmospheres falls into two chemical categories: Titan (Krasnopolsky, 2010; Lavvas et al., 2008a,b; Wilson and reducing and oxidizing. A redox (reduction–oxidation) state Atreya, 2004), and probably the early Earth (Pavlov et al., indicates the chemical character of atmospheres, for example, 2001; Wolf and Toon, 2010; Zerkle et al., 2012). whether elements such as carbon are likely to be found in their In addition to the atmospheres discussed in the preceding reduced form (methane, CH4) or oxidized variety (carbon text, some smaller bodies possess very thin atmospheres. Exo- dioxide, CO2). spheres exist directly above the surfaces of several moons, The solar system contains several examples of oxidizing and which are defined as atmospheric layers of such low density reducing atmospheres. Reducing atmospheres are those that that they are virtually collisionless. We find the following thin are relatively rich in reducing gases, typically hydrogen-bearing atmospheres, where the surface pressure is given in bar gases, such as hydrogen itself (H ), methane (CH ), and pos- (1 bar 105 Pa) in parentheses: 2 4 ¼ sibly ammonia (NH3). Examples include all the giant planets and Saturn’s largest moon, Titan (Table 1).
Details
-
File Typepdf
-
Upload Time-
-
Content LanguagesEnglish
-
Upload UserAnonymous/Not logged-in
-
File Pages44 Page
-
File Size-