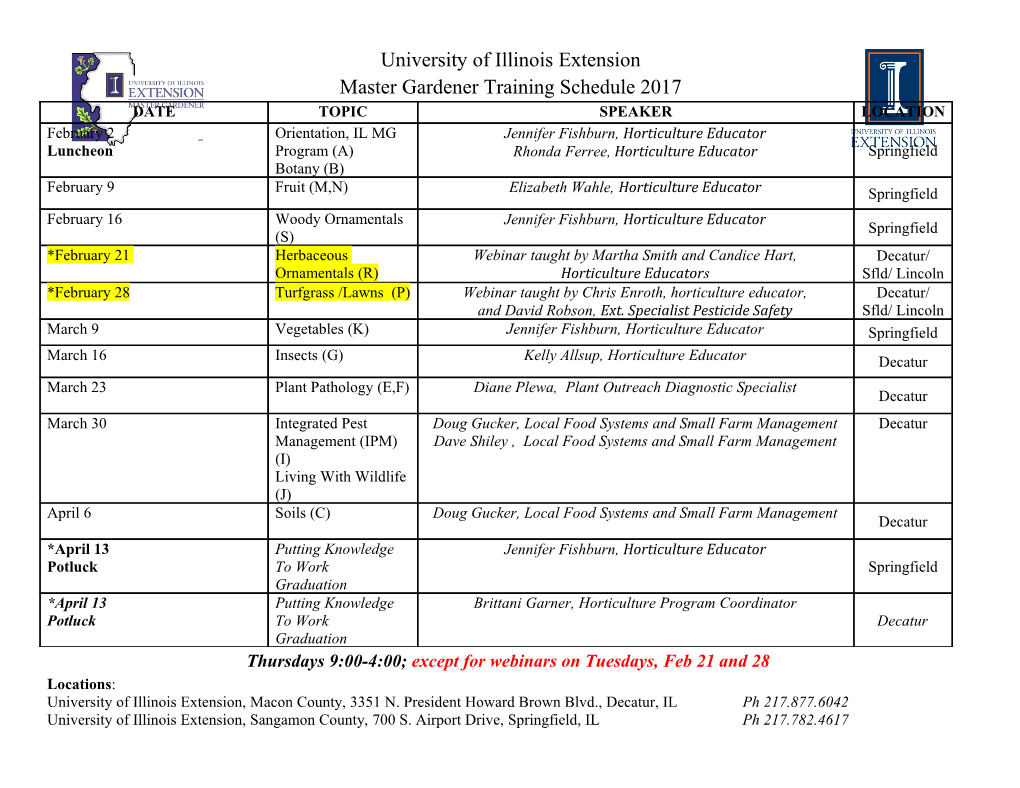
PUBLICATIONS Global Biogeochemical Cycles RESEARCH ARTICLE Climatological distribution of aragonite 10.1002/2015GB005198 saturation state in the global oceans The scientific results and conclusions, as Li-Qing Jiang1, Richard A. Feely2, Brendan R. Carter3, Dana J. Greeley2, Dwight K. Gledhill4, well as any views or opinions expressed 5 herein, are those of the authors and do and Krisa M. Arzayus fl not necessarily re ect the views of 1 NOAA or the Department of Commerce. Cooperative Institute for Climate and Satellites, Earth System Science Interdisciplinary Center, University of Maryland, College Park, Maryland, USA, 2Pacific Marine Environmental Laboratory, National Oceanic and Atmospheric Administration, Seattle, Washington, USA, 3Joint Institute for the Study of the Atmosphere and Ocean, University of Washington, Seattle, Key Points: 4 fi • Washington, USA, Ocean Acidi cation Program, National Oceanic and Atmospheric Administration, Silver Spring, Climatological aragonite saturation in 5 surface and subsurface global oceans Maryland, USA, National Centers for Environmental Information, National Oceanic and Atmospheric Administration, Silver are presented Spring, Maryland, USA • Mechanisms controlling aragonite saturation state distributions are discussed Abstract Aragonite saturation state (Ωarag) in surface and subsurface waters of the global oceans was • Subannual and decadal changes of aragonite saturation state are calculated from up-to-date (through the year of 2012) ocean station dissolved inorganic carbon (DIC) and presented total alkalinity (TA) data. Surface Ωarag in the open ocean was always supersaturated (Ω > 1), ranging between 1.1 and 4.2. It was above 2.0 (2.0–4.2) between 40°N and 40°S but decreased toward higher latitude to below 1.5 fl Supporting Information: in polar areas. The in uences of water temperature on the TA/DIC ratio, combined with the temperature effects • – Tables S1 S9 on inorganic carbon equilibrium and apparent solubility product (K′sp), explain the latitudinal differences in surface Ωarag. Vertically, Ωarag was highest in the surface mixed layer. Higher hydrostatic pressure, lower water Correspondence to: temperature, and more CO2 buildup from biological activity in the absence of air-sea gas exchange helped L.-Q. Jiang, Ω [email protected] maintain lower arag in the deep ocean. Below the thermocline, aerobic decomposition of organic matter along the pathway of global thermohaline circulation played an important role in controlling Ωarag distributions. Seasonally, surface Ωarag above 30° latitudes was about 0.06 to 0.55 higher during warmer months than during Citation: Ω Jiang, L.-Q., R. A. Feely, B. R. Carter, colder months in the open-ocean waters of both hemispheres. Decadal changes of arag in the Atlantic and D. J. Greeley, D. K. Gledhill, and PacificOceansshowedthatΩarag in waters shallower than 100 m depth decreased by 0.10 ± 0.09 (À0.40 À K. M. Arzayus (2015), Climatological ± 0.37% yr 1) on average from the decade spanning 1989–1998 to the decade spanning 1998–2010. distribution of aragonite saturation state in the global oceans, Global Biogeochem. Cycles, 29, 1656–1673, doi:10.1002/2015GB005198. 1. Introduction Received 29 MAY 2015 The global oceans have performed a substantial climate service by taking up ~30% of the anthropogenic Accepted 15 SEP 2015 carbon dioxide (CO ) emissions over the past 200 years [Canadell et al., 2007]. Based on emission data from Accepted article online 18 SEP 2015 2 Published online 13 OCT 2015 2004 to 2013, CO2 released from the burning of fossil fuels, cement production, and land use changes À1 15 15 accounts for a total of 36 GtCO2 yr (1 GtCO2 =1×10 gofCO2 = 0.27 × 10 g of carbon). Of the CO2 À1 À1 emitted during the last decade, about 16 GtCO2 yr (44%) stayed in the atmosphere, and 9 GtCO2 yr (26%) entered the global oceans [Le Quéré et al., 2014]. The balance is assumed to be taken up by the terrestrial biosphere. 2À Ocean CO2 uptake lowers ocean pH, carbonate ion (CO3 ) concentrations, and calcium carbonate (CaCO3) saturation state in the global oceans. This process is commonly referred to as “ocean acidification (OA)” [Broecker and Clark, 2001; Caldeira and Wickett, 2003; Feely et al., 2004a; Orr et al., 2005]. OA impacts ecosystems by making it more difficult for calcifying organisms such as corals, mollusks, and some planktonic organisms to form shells and other hard parts and by making these structures more vulnerable to dissolution [Doney et al., 2009; Gattuso and Hansson, 2011; Bednaršek et al., 2012, 2014]. The saturation state of carbonate minerals is defined by Âà ½ÂCa2þ CO2- Ω ¼ 3 ′ (1) Ksp 2+ 2À where Ω is the saturation state (Ω > 1 favors precipitation and Ω < 1 favors dissolution), [Ca ] and [CO3 ] Published 2015. American Geophysical are the calcium and carbonate ion concentrations, and K′sp is the apparent solubility product. The saturation Union. This article is a US Government work and, as such, is in the public states of aragonite and calcite (two of the most common calcium carbonate minerals in the ocean) dropped domain in the United States of America. to ~84% of preindustrial values by the year 2000 and are expected to decrease to ~50% of preindustrial JIANG ET AL. ARAGONITE SATURATION CLIMATOLOGY 1656 Global Biogeochemical Cycles 10.1002/2015GB005198 Figure 1. Information for the sampling stations that were used for this study: (a) number of sampling stations that were collected in a specific year and (b) spatial distributions of sampling stations. The color indicates the sampling year. values by 2100 under the IS92a emission scenario of the Intergovernmental Panel on Climate Change report 1995 [Wolf-Gladrow et al., 1999; Feely et al., 2009b]. Surface aragonite and calcite saturation state distributions were studied by Feely et al. [2009a], using data from the Global Ocean Data Analysis Project (GLODAP) Version 1.1 (which includes data measured before 1999) [Key et al., 2004]. Takahashi et al. [2014] calculated surface aragonite and calcite saturation states (nor- malized to the year 2005) using partial pressure of carbon dioxide (pCO2) data from the Lamont Doherty Earth Observatory database and total alkalinity (TA) estimated from sea surface salinity. However, the distributions of aragonite saturation states in subsurface waters have not been described in detail in the earlier studies. We use additional data collected since the GLODAP version 1.1 release to reexamine the global aragonite saturation state. During this decade, ocean station data with multiple carbon parameters have nearly doubled (Figure 1a). Here we map and describe both surface and subsurface Ωarag distributions in the global oceans, discuss the mechanisms controlling the Ωarag distribution and seasonality, and estimate decadal Ωarag changes in the major ocean basins. 2. Methods Data for this study were obtained from the Global Ocean Data Analysis Project (GLODAP) [Key et al., 2004], the Carbon Dioxide in the Atlantic Ocean (CARINA) [Key et al., 2010], the Pacific Ocean Interior Carbon (PACIFICA) [Suzuki et al., 2013], and some recent cruise data sets [Feely and Sabine, 2011; Wanninkhof et al., 2014]. Duplicated stations—defined as stations with identical longitude, latitude, and sampling date—were JIANG ET AL. ARAGONITE SATURATION CLIMATOLOGY 1657 Global Biogeochemical Cycles 10.1002/2015GB005198 removed. Data with a salinity value of less than 25 (a total of about 30 stations) were excluded since most were measured in river plumes and do not reflect the open ocean. A total of 11,431 stations were selected for this study (Figure 1). Ωarag was calculated using a MATLAB version [van Heuven et al., 2009] of the CO2SYS program [Lewis and Wallace, 1998] based on equation (1). [Ca2+] in seawater was assumed to be conservative with salinity according to Millero [1995]: Âà Ca2þ ¼ 293:86 S (2) 2+ À1 2À where the unit of [Ca ]isμmol kg , and S is salinity. [CO3 ] was calculated from in situ temperature, pressure, salinity, dissolved inorganic carbon (DIC), total alkalinity (TA), silicate and phosphate with the À dissociation constants for carbonic acid of Lueker et al. [2000], potassium bisulfate (KHSO4 )ofDickson [1990a], boric acid of Dickson [1990b], and the total borate concentration equations of Lee et al. [2010]. The apparent solubility product (K′sp) of aragonite was calculated from temperature and salinity with the equation of Mucci [1983]. For surface waters at ambient pressure, ′ ¼ –½ðÞ– : – : þ : = þ : pKsp 171 945 0 077993 T 2903 293 T 71 595 logT (3) þ ðÞ–0:068393 þ 0:0017276 T þ 88:135=T S0:5– 0:10018 S þ 0:0059415 S1:5 where pK′sp = Àlog K′sp, and T is temperature in kelvin (K). The pressure dependence of K′sp is formulated in terms of the change in molal volume and compressibility as described in Millero [1995] using the corrected constants as presented in Appendix A.11 of Zeebe and Wolf-Gladrow [2001]: n ′ P ¼ ′ 0 – : ––½þðÞþ : ðÞ– : = ðÞÂðÞ= : pK sp pKsp 0 434 hio46 0 5304 T 273 15 RT P 10 0 5 (4)  ðÞ–0:011760 þ 0:0003692 ðÞT–273:15 = ðÞÂRT ðÞP=10 2 where the subscripts “0” and “P” on the solubility products denote conditions at the surface and at pressure P À (dbar), respectively. The ideal gas constant R is 83.13 cm3 bar (mol K) 1. More details of the saturation state calculation can be found in Wanninkhof et al. [2015]. Using the error propagation methods described in Taylor [1997], uncertainties of the calculated [Ca2+], 2À [CO3 ], and K′sp were estimated to be 0.06%, 3.60%, and 0.00%, respectively. However, for K′sp, the uncer- tainty is primarily from the use of an empirical equation to reflect the real-world K′sp, rather than from propa- gating the uncertainties of T, S and P to the result of the equation of Mucci [1983]. According to Mucci [1983], the uncertainty of the former is much larger at about 5%.
Details
-
File Typepdf
-
Upload Time-
-
Content LanguagesEnglish
-
Upload UserAnonymous/Not logged-in
-
File Pages18 Page
-
File Size-