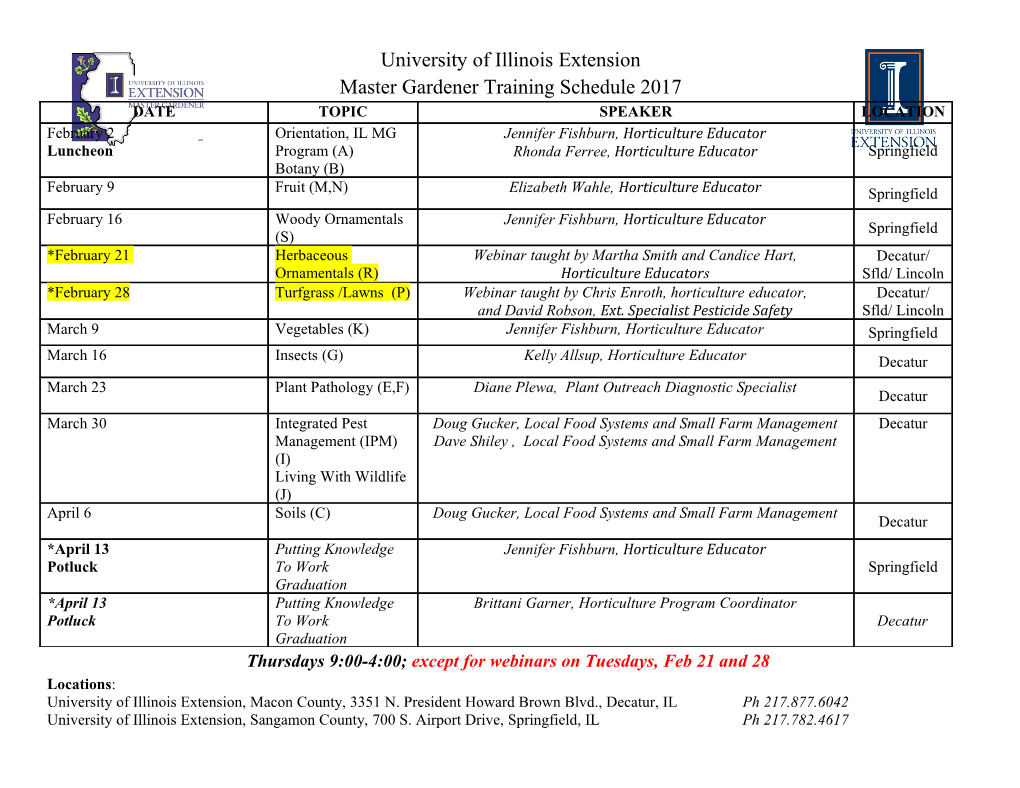
Stellarators and linear devices Gábor Veres/Gergő Pokol BME NTI Fusion devices 24. February 2020 Ingredients of fusion Confinement and Stability 2 Confinement (force-free equilibrium) + Stability („rigidity” against perturbations) 3 Linear devices I 4 The magnetic mirror In slowly varying magnetic fields the constant magnetic moment is constant. 푣0 If the initial parallel velocity of the 푣⊥ particle is too large, the particle escapes 푣∥ 5 Linear devices II 6 Linear devices III (Magneto-Optical Traps, MOT) anti-Helmholtz coils and Ioffe-Pritchard trap twisted metal bars to achieve 3D trapping The magnetic field B, versus position 7 The Bennett-pinch (Z-pinch) 1D (linear) equilibrium The simplest equilibrium is the Bennett-pinch (Z-pinch) Why it does not work?: Suffers from numerous, violent instabilities 8 The theta-pinch 1D (linear) equilibrium This equilibrium is stable, but transient. 9 The screw-pinch 1D (linear) equilibrium This equilibrium is stable, but end-losses are substantial 10 Linear devices summary Problems: Loss cones at the ends Instabilities Proposed solutions: - Tandem mirror (electrostatic boundary) - Plasma rotation - Multiple mirrors (diffusive losses) around the axis - ICRH at ends (empty loss cones) - Inverse curved - strong NBI heating (non-thermal population) regions - long device (~100 m) 11 2D (toroidal) equilibrium Partcle drifts in electrical and inhomogeneous magnetic fields: This leads to an outward drift electron gradB-drift Rotational transform (= helical magnetic field) is necessary E ExB-drift ion gradB-drift 12 Rotational transform (iota) 휗 휄 = lim 푛 푛→∞ 2휋푛 2휋 푞 = 휄 The safety factor for tokamaks 13 ZETA Reactor (UK) „Zero Energy Thermonuclear Assembly” – from 1957 14 Plasma balls: 3D equilibrium No „self-confinement” in 3D!!!! 15 Some history (fake news I) Ronald Richter and the Huemul Project (1951), Thermotron → a complete fiasco 16 Some history (fake news II) 17 The helical field is necessary, but not sufficient Vertical field + strong toroidal field to supress instabilities 18 Stellarator zoology 19 Stellarator history Lyman Spitzer with his Model-A stellarator in 1958 20 Stellarator history - Princeton Model-B stellarator T 1 2n D ~ B2 1 kT D B 16 eB Model C stellarator 21 Wendelstein stellarators Wendelstein I-A (1961): „Racetrack” geometry Major radius: 35 cm Minor radius: 2 cm Magnetic field: 1 T Ohmic heating l=3 winding Wendelstein I-B l=2 winding22 Classical stellarator 23 Wendelstein stellarators Wendelstein II-A (1968): Torus geometry Major radius: 50 cm Minor radius: 5 cm Magnetic field: 0,6 T RF heating 24 Classical stellarator – WII-A 25 Wendelstein stellarators Wendelstein II-B (1971): Torus geometry Major radius: 50 cm Minor radius: 5 cm Magnetic field: 1,25 T RF heating OH transformer 26 Confinement depends on iota Let’s design the iota-profile! = 1/q Ratios of small rational numbers are infavourable, because field line close on themselvs after a few turns 27 Modular stellarator 28 (Advanced) Wendelstein stellarators Wendelstein 7-A (1976): Torus geometry Major radius: 2 m Minor radius: 0,1 m Magnetic field: 3,4 T Hydrogen plasma Neutral beam heating 29 Wendelstein stellarators Wendelstein 7-AS (1988): Modular stellarator (5 modules) Partially optimized Major radius: 2 m Minor radius: 0,18 m Magnetic field: 2,5 T Hydrogen and deuterium plasma 30 W7-AS 31 Wendelstein stellarators WEGA (1970 2001 2013): Torus geometry Major radius: 0,72 m Minor radius: 0,11 m Magnetic field: 0,9 T Hydrogen plasma Educational purpose From 2014: Hybrid Illinois Device for Research and Applications 32 Magnetic geometry 33 Island divertor 34 W-7x stellarator (full 3D) 35 Wendelstein stellarators 1970 1980 1990 2000 2010 W7-X Greifswald Wega Garching München Wendelstein 7-AS W 7-A W 2-B W 2-A W1-B Wendelstein line stellarators W 1-A 36 Modular stellarator – HSX 37 Heliotron 38 Heliotron – LHD 39 Heliotron – LHD 40 Torsatron 41 Torsatron – TJ-K 42 Heliac 43 Heliac – TJ-II 44 Heliac – TJ-II 45 Stellarator magnetic fields 46 Sources 1. J.L. Johnson: The evolution of stellarator theory st Princeton, PPPl-3629 (2001) 2. H. Wobig et al.: Stellarator research at the IPP Garching, IPP report (2002) 3. M. Hirsch et al.: Major results form the stellarator Wendelstein 7-AS, Plasma Physics and Controlled Fusion, 50, 053001 (2008) 4. B.A. Carreras et al.: Progress in strellarator/heliotron research:1981-1986, Nuclear Fusion, 28, 1613 (1988) 5. http://npre.illinois.edu/news/hidra%E2%80%99s-many-uses 6. http://www.hsx.wisc.edu/ 7. http://www.lhd.nifs.ac.jp/en/lhd/ 8. http://www.igvp.uni-stuttgart.de/forschung/projekte-pd/tjk.en.html 9. http://fusionsites.ciemat.es/tj-ii-2/ 10.Stellarator News: http://web.ornl.gov/sci/fed/stelnews/ 47.
Details
-
File Typepdf
-
Upload Time-
-
Content LanguagesEnglish
-
Upload UserAnonymous/Not logged-in
-
File Pages47 Page
-
File Size-