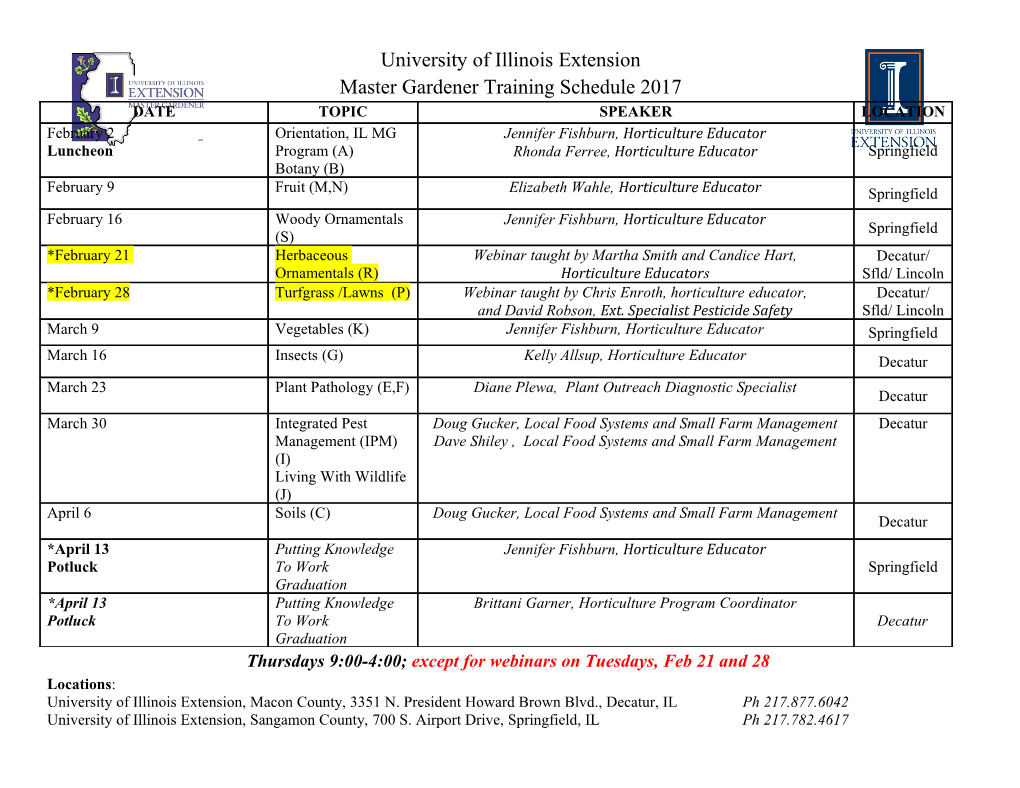
An investigation into the parasitic barnacle, Anelasma squalicola; prevalence, infection behaviour and effects on its host, Etmopterus spinax, in Lusterfjord, Norway. Photo by Rees et al., 2014 By Lasse Eliassen Supervised by Henrik Glenner, Arne Skorping and Knut Helge Jensen University of Bergen Abstract Anelasma squalicola is a recently evolved parasite. Very little is known of its biology due to its normally low prevalence in deep sea lantern sharks (family: Etmopteridae), in which it is found embedded in the skin. A population of more heavily infected sharks (Etmopterus spinax) in Lusterfjord, Norway, has allowed for sampling by trawl, and subsequent observations and measurements of the parasite and host. The study shows that E. spinax has a heterogeneous population structure and potentially narrow home range, which likely affects prevalence and dispersal of A. squalicola. A. squalicola appears capable of infecting hosts regardless of size. It has high site specificity, which may be due to areas of the shark where the skin is easier to penetrate. It is most commonly found in pairs, which gives it an atypical intensity distribution. The data suggests the first individual to settle can attract partners to the same site, but this attraction does not result in more infections at other sites on the host. Attracting more than one partner appears to severely reduce their fecundity, which may imply a crowding effect. Infection did not affect liver mass or condition of hosts, but appears to prevent maturation in males. This study provides the most extensive parasitological description of A. squalicola to date, and reveals both a complex host population as well as a highly distinctive infection behaviour, which combined shape this parasite-host interaction. Table of contents 1. Introduction 1.1. Description and history of A. squalicola………………………………………………. 1-5 1.2. Aims……………………………………………………………………………………... 5-7 2. Methods: 2.1. Species taxonomy……………………………………………………………………...... 8 2.2. Trawling…………………………………………………………………………………. 9-12 2.3. Observations and measurements……………………………………………………… 13-17 2.4. Statistical procedures……………………………………………………………..……. 18 3. Result 3.1. Stations ………………………………………………………………………………….. 19-20 3.2. Parasite and host size distributions……………………………………………………. 21-22 3.3. Observations: intensity, site and egg presence…………………………………………23-26 3.4. Various analyses 3.4.1. Is A. squalicola able to infect hosts regardless of host size ............................. 27 3.4.2. Are partners similar in size? ............................................................................. 28-29 3.4.3. Maturity in A. squalicola …………………………………………………….. 30 3.4.4. Which factors affect egg presence in A. squalicola? ………………………… 31 3.4.5. Site specificity: does site affect size or fecundity? .......................................... 32-33 3.4.6. Intraspecific competition in A. squalicola …………………………………… 34 3.5. Effects on host 3.5.1. Does infection affect host susceptibility?.......................................................... 35-36 3.5.2. Hepatosomatic Index........................................................................................ 37 3.5.3. Fulton‟s condition factor ……………………………………………………. 38 3.5.4. Does A. squalicola impact host reproduction? …………………………….... 39-40 4. Discussion 4.1. Summary of results …………………………………………………………………….. 41 4.2. Prevalence and geographic distribution of A. squalicola …………………………….. 42-49 4.3. The infection biology of A .squalicola ………………………………………………… 50-55 4.4. Intraspecific competition in A. squalicola …………………………………………….. 56-58 4.5. The potential for evolution of male dwarfism ………………………………………… 59 4.6. Effects on host 4.6.1. Condition factor and hepatosomatic index …………………………………... 60 4.6.2. Reduced fecundity of host …………………………………………………… 61 5. Conclusion ………………………………………………………………………………………………………………. 64 6. Acknowledgement ………………………………………………………………………………………………………. 65 7. References ……………………………………………………………………………………………………………….. 66-73 8. Glossary ………………………………………………………………………………………………………………….. 73 9. Appendix ………………………………………………………………………………………………………………… 74-87 1 1. Introduction 1.1. Description and history of A. squalicola A. squalicola is a monophyletic, stalked barnacle found on certain deep sea sharks of the family Etmopteridae. It is found partially embedded in the tissue of the shark, into which it extends a system of rootlets from its peduncle; an organ normally used for attaching to the substratum. The curious morphology of A. squalicola has puzzled scientists, including Charles Darwin (Darwin, 1851). Conventional barnacles are suspension feeders; they rely on filtering minute planktonic organisms. This has led to the evolution of cirri („legs‟) with very fine setae (hairs) used to catch food. As follows, they also have a mouth and a digestive system used to masticate and process their food. In contrast to conventional barnacles, the filter-feeding organs, and indeed the general morphology of A. squalicola, do not appear to allow for suspension feeding: the cirri, which Darwin describes as “shapeless and rudimentary” (Darwin, 1851), are devoid of setae and appear unable to catch food items, and the mouth is reduced in size. How it obtains nutrients has therefore been a key question. Despite the unusual morphology of A. squalicola, it has rarely been studied. The first person to write about it was the Norwegian naturalist Gunnerus (1758), who correctly identified it as a crustacean. However, his focus at the time was on the shark and not the attached barnacle, so no further effort was made to explore its biology. Because Gunnerus published his findings in a rather obscure journal, his work remained unnoticed for decades (Broch 1919). Nearly a century later, the Swedish zoologist Lovén (1844) identified the organism as a barnacle and 2 described it as Alepas squalicola. Later, working on his Monograph on Cirripedia, Darwin realised that Lovén had placed it in the wrong genus and re-described the species into a new monotypic genus, A. squalicola (Darwin 1851). In his Mongraph on Cirripedia (Darwin, 1851), Darwin hypothesised that the rootlets are mainly a means of anchoring to the host, and concluded that “[A. squalicola] can reach minute animals crawling by on the surface of the shark’s body”. In fact, he quite extensively described A. squalicola as a predator, including how the mouthparts are “beautifully adapted to catch and force down any small living creature into the muscular oesophagus”. Despite not finding any food present in the gut of the specimen, Darwin did not consider that this barnacle could have a completely different means of obtaining nutrients. In his defence, Darwin only had one specimen available to him, and at the time did not know of the existence of parasitic barnacles, which he was introduced to later through the work on rhizocephalan barnacles by German zoologist Müller (1862). If he had known of the root system in rhizocephalan barnacles, Darwin would probably have made the link between those roots and the rootlets in A. squalicola, and seen its potential as a parasite. Today, the rootlets are believed to have evolved in order to increase the surface area of the peduncle, which at a pivotal point in time evolved the ability to absorb nutrients from the shark‟s tissue. Although there has been some uncertainty about the extent to which A. squalicola is a true parasite or not, with some describing it as a facultative “meso” parasite (Yano & Musick, 2000), a recent study by Ommundsen et al. (2016) strongly support the obligatory parasitic nature of the species. From their investigation they found that none of the inspected specimens had food in their guts; their mouthparts were highly reduced, with severe left-to-right asymmetry; and the cirri were highly 3 abnormal and lacking the setae necessary for filtering. The degenerate nature of the organs associated with filter-feeding thus suggests a lack of stabilising selection, implying that the precise morphology needed in order to filter feed is no longer of importance to the survival of A. squalicola. Isotopic δ13Carbon and δ15Nitrogen analyses further revealed that the trophic level was more similar to that of a parasite than a conventional barnacle (Ommundsen et al., 2016). The continuing presence of the conventional filter-feeding traits alongside the rather limited parasitic root system, are difficult to explain unless viewed in the light of evolution. This overlapping presence of traits for two different modes of feeding suggests that the evolutionary transition to parasitism occurred very recently. This assumption is further backed up by the limited genetic differentiation between the Cytochrome Oxidase 1 genes of individuals from distant regions of the world (New Zealand, South Africa, the southern and western Atlantic and Sognefjorden) (Rees et al., 20014). Phoresy, the act of hitching a ride on another organism, is thought to facilitate the evolution of parasitic relationships (Poulin, 2007). Phoresy is common within Cirripedia, with barnacles found on various marine mammals, such as sea cows (Manatees) and whales, as well as on reptiles like turtles or sea snakes. Given A. squalicola‟s parasitic association with a vertebrate host, one might postulate that it came from a lineage of epibionts, especially on vertebrates, such as whale barnacles (Balanomorpha: Coronulidae). However, Darwin (1851) dismissed this relationship due to A. squalicola‟s morphology, in particular the peduncle, which is not found in balanomorphs. 4 Recent
Details
-
File Typepdf
-
Upload Time-
-
Content LanguagesEnglish
-
Upload UserAnonymous/Not logged-in
-
File Pages90 Page
-
File Size-