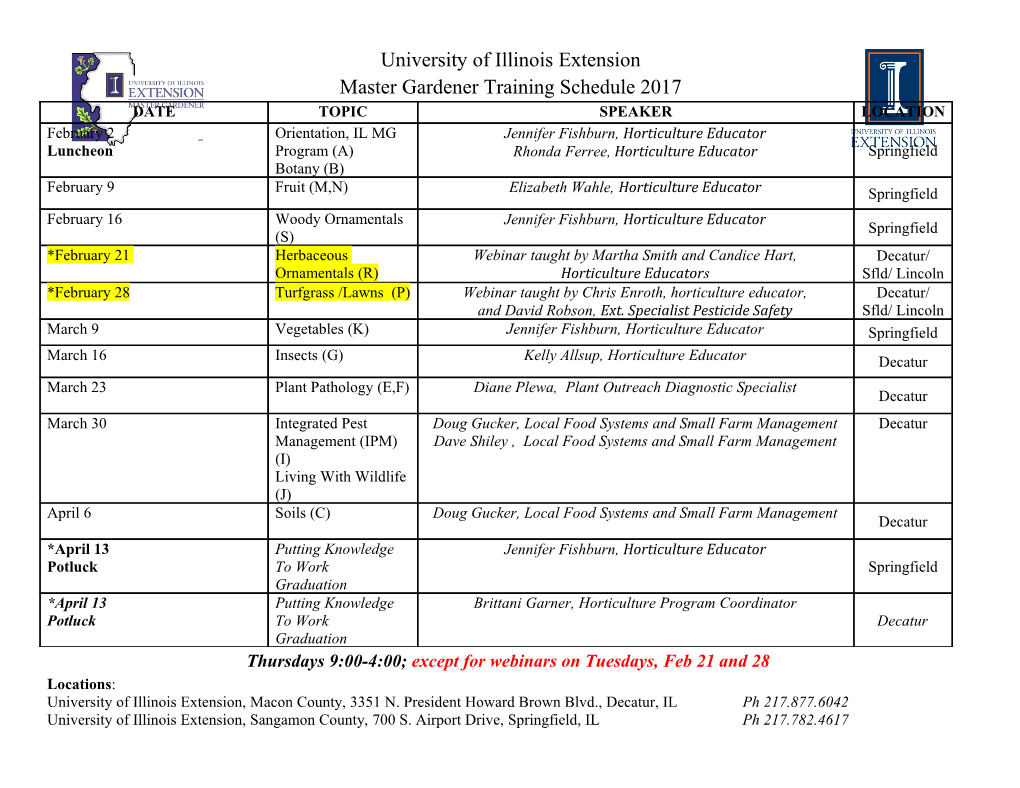
Author’s Accepted Manuscript In vitro metabolic engineering for the production of α-ketoglutarate Barbara Beer, André Pick, Volker Sieber www.elsevier.com/locate/ymben PII: S1096-7176(16)30293-2 DOI: http://dx.doi.org/10.1016/j.ymben.2017.02.011 Reference: YMBEN1225 To appear in: Metabolic Engineering Received date: 30 December 2016 Revised date: 16 February 2017 Accepted date: 21 February 2017 Cite this article as: Barbara Beer, André Pick and Volker Sieber, In vitro metabolic engineering for the production of α-ketoglutarate, Metabolic Engineering, http://dx.doi.org/10.1016/j.ymben.2017.02.011 This is a PDF file of an unedited manuscript that has been accepted for publication. As a service to our customers we are providing this early version of the manuscript. The manuscript will undergo copyediting, typesetting, and review of the resulting galley proof before it is published in its final citable form. Please note that during the production process errors may be discovered which could affect the content, and all legal disclaimers that apply to the journal pertain. In vitro metabolic engineering for the production of α-ketoglutarate Barbara Beera, André Picka, Volker Siebera,b* aChair of Chemistry of Biogenic Resources, Technical University of Munich, Schulgasse 16, 94315 Straubing, Germany bCatalysis Research Center, Technical University of Munich, Ernst-Otto-Fischer-Str. 1, 85748 Garching, Germany *Corresponding author. Prof. Dr. Volker Sieber, Technical University of Munich, Schulgasse 16, 94315 Straubing, Germany, [email protected] Abstract α-Ketoglutarate (aKG) represents a central intermediate of cell metabolism. It is used for medical treatments and as a chemical building block. Enzymatic cascade reactions have the potential to sustainably synthesize this natural product. Here we report a systems biocatalysis approach for an in vitro reaction set- up to produce aKG from glucuronate using the oxidative pathway of uronic acids. Because of two dehydrations, a decarboxylation, and reaction conditions favoring oxidation, the pathway is driven thermodynamically towards complete product formation. The five enzymes (including one for cofactor recycling) were first investigated individually to define optimal reaction conditions for the cascade reaction. Then, the kinetic parameters were determined under these conditions and the inhibitory effects of substrate, intermediates, and product were evaluated. As cofactor supply is critical for the cascade reaction, various set-ups were tested: increasing concentrations of the recycling enzyme, different initial NAD+ concentrations, as well as the use of a bubble reactor for faster oxygen diffusion. Finally, we were 1 able to convert 10 g/L glucuronate with 92% yield of aKG within 5 h. The maximum productivity of 2.8 g L−1 h−1 is the second highest reported in the biotechnological synthesis of aKG. Abbreviations: AbC, ammonium bicarbonate; aKG, α-ketoglutarate; GlucD, glucarate dehydratase; Kdg, 5- keto-4-deoxyglucarate; KdgD, Kdg dehydratase; Kgsa, α-ketoglutaric semialdehyde; KgsalDH, Kgsa dehydrogenase; KP, potassium phosphate; NOX, NADH oxidase; UDH, uronate dehydrogenase Keywords: In vitro reaction, enzyme cascade reaction, α-ketoglutarate, bubble reactor, systems biocatalysis 1. Introduction α-Ketoglutaric acid (aKG) is an important intermediate of the tricarboxylic acid cycle and amino acid metabolism. Additionally, aKG plays a central role in nitrogen assimilation and storage. Besides its importance in cell metabolism, it is of particular industrial interest due to its broad range of applications, namely, as a dietary supplement, component of infusion solutions or wound healing compounds, and as a building block for the chemical synthesis of heterocycles (Chernyavskaya et al., 2000; Huang et al., 2006; Stottmeister et al., 2005; Verseck et al., 2009). D-glutamic acid, a pharmaceutical for bowel disorder, can be produced from aKG using D-aminotransferases (Taylor et al., 1998). In addition, it is used together with 5-hydroxymethylfurfural for the production of an agent that protects humans and animals from oxidative stress by increasing the antioxidant capacity (Moser et al., 2007). A potential application in biomedicine was described by Barrett and Yousaf (2008). By thermal polycondensation of aKG and one of the triols, glycerol, 1,2,4-butanetriol, or 1,2,6-hexanetriol, elastomers [poly(triol-α-ketoglutarate)] with a wide range of mechanical and chemical properties were provided, which could potentially be used for tissue engineering or drug delivery. Currently, aKG is chemically synthesized from succinic acid and oxalic acid diethyl esters with cyanohydrines with a yield of 75% or by hydrolysis of acyl cyanides (Stottmeister et al., 2005). These multi-step synthetic processes have numerous disadvantages, such as the use of hazardous chemicals 2 (e.g., cyanides), generation of toxic waste, presence and disposal of a catalyst containing copper, or low product selectivity due to the formation of various side products such as glycine and other organic acids (Cooper et al., 1983; Evans and Wiselogle, 1945; Stottmeister et al., 2005; Verseck et al., 2009). Biocatalysis has emerged as an alternative to chemical synthesis due to reduced environmental pollution and the availability of different starting materials. So far, the focus for aKG production has been on fermentation-based techniques. In particular, the yeast Yarrowia lipolytica has been studied and metabolically engineered for the production of aKG since the 1960s (Otto et al., 2011; Tsugawa et al., 1969a; Tsugawa et al., 1969b; Tsugawa and Okumura, 1969). Although great progress has been made in recent decades, cultivation conditions to achieve aKG overproduction have to be adjusted very carefully with respect to thiamine and nitrogen concentration, pH, and aeration (Guo et al., 2014; Otto et al., 2011; Yovkova et al., 2014). Nonetheless, by-products, especially pyruvate and other organic acids, hamper the industrial production of aKG using a whole-cell catalyst (Guo et al., 2014; Holz et al., 2011; Otto et al., 2012). These disadvantages of cell-based synthesis, namely, a large number of metabolic pathways with many options to adjust and alter without knowledge of the consequences for the cell’s energetics, metabolic bypasses, or improvement of product formation (Petzold et al., 2015; Zadran and Levine, 2013), are not new. Therefore, many researchers have focused on in vitro metabolic pathways, also called “systems biocatalysis” (Fessner, 2015; Guterl et al., 2012; Krutsakorn et al., 2013; Myung et al., 2014; Tessaro et al., 2015; Zhu and Zhang, 2016) Here, the reaction route can be set up according to natural metabolic pathways, but it can also be completely artificial. The simpler the pathway, the more easily it can be adjusted and improved. Therefore, the number of enzymes, mandatory cofactors for enzyme activity, and cofactor balance as well as thermodynamic equilibrium of the reaction have to be considered, when developing an in vitro metabolic pathway. During route scouting, the substrate also plays a critical role. It should be readily available, cheaper than the product, and, with the goal of making industrial processes more sustainable, derived from a renewable resource. Among the vast number of natural metabolic pathways, the oxidative pathway of C6 uronic acids holds potential for α-ketoglutarate synthesis in an in vitro-system. The number of enzymes involved is low (four enzymes), only one cofactor is needed (NAD+) and the substrate could be obtained from industrial wastes of renewable resources. The pathway has been identified in various Pseudomonas species and Agrobacterium tumefaciens (Aghaie et al., 2008; Andberg et al., 2012) and is similar to an alternative 3 utilization of C5-carbohydrate as identified for xylose, arabinose, and lyxonate (Stephens et al., 2007; Watanabe et al., 2006a; Watanabe et al., 2006b). C6 uronic acids such as glucuronate and galacturonate are omnipresent sugar derivatives. They constitute monomeric building blocks of structural polysaccharides such as hemicellulose and pectin (Saha, 2003). They are also present in seaweed macroalgae and microalgae, which are considered as feedstock of third-generation biorefineries (Jung et al., 2013; Lahaye and Robic, 2007). Additionally, they are components of extracellular polymeric substances responsible for microbial biofilm formation (Sutherland, 2001). The pathway toward aKG includes the following steps: First, the uronic acid, namely, glucuronic acid, is oxidized by an NAD+-dependent uronate dehydrogenase (UDH) to yield glucarate. The aldaric acid is dehydrated to 5-keto-4-deoxyglucarate (Kdg), which is then converted by Kdg dehydratase, a decarboxylating dehydratase that gives α-ketoglutaric semialdehyde (Kgsa). The final step is another NAD+-dependent oxidation by α-ketoglutaric semialdehyde dehydrogenase (KgsalDH). The thermodynamic equilibrium of the reaction strongly favors the product due to the irreversible decarboxylation and the essentially irreversible oxidation of two aldehyde groups to carboxylic acids. To balance the NAD+ consumption in an in vitro system, an NADH oxidase, preferably a water-forming one, has to be included in the process (Kroutil et al., 2004; Nowak et al., 2015; Ödman et al., 2004). In total, five enzymes, one cofactor, and a catalytic amount of Magnesium-ions are necessary to convert the renewable resource glucuronic acid to the industrially relevant compound α-ketoglutarate. We investigated whether this
Details
-
File Typepdf
-
Upload Time-
-
Content LanguagesEnglish
-
Upload UserAnonymous/Not logged-in
-
File Pages27 Page
-
File Size-